- 1College of Forestry, Northwest A&F University, Xianyang, China
- 2Boone Pickens School of Geology, Oklahoma State University, Stillwater, OK, United States
- 3Guangdong Laboratory for Lingnan Modern Agriculture, College of Forestry and Landscape Architecture, South China Agricultural University, Guangzhou, China
Introduction: Saline-alkali stress seriously endangers the normal growth of Populus simonii×P. nigra. Arbuscular mycorrhizal (AM) fungi can enhance the saline-alkali tolerance of plants by establishing a symbiotic relationship with them.
Methods: In this study, a pot experiment was conducted to simulate a saline-alkali environment where Populus simonii×P. nigra were inoculated with Funneliformis mosseae to explore their effects on the saline-alkali tolerance of Populus simonii×P. nigra.
Results and Discussion: Our results show that a total of 8 NHX gene family members are identified in Populus simonii×P. nigra. F. mosseae regulate the distribution of Na+ by inducing the expression of PxNHXs. The pH value of poplar rhizosphere soil is reduced, result in the promote absorption of Na+ by poplar, that ultimately improved the soil environment. Under saline-alkali stress, F. mosseae improve the chlorophyll fluorescence and photosynthetic parameters of poplar, promote the absorption of water, K+ and Ca2+, thus increase the plant height and fresh weight of aboveground parts, and promote the growth of poplar. Our results provide a theoretical basis for further exploring the application of AM fungi to improve the saline-alkali tolerance of plants.
1 Introduction
About 1×109 hm2 of saline-alkali soil is distributed all over the world (Liu et al., 2022), which seriously restricts the development of agricultural economy. Influenced by natural factors such as climate change and human factors such as improper irrigation methods, the saline-alkali region continues to expand from year to year (Evelin et al., 2019). China’s existing saline-alkali land is about 3.6×107 hm2, mainly distributed in the northeast, northwest and coastal areas of north China (Lim et al., 2017; Yang et al., 2019b). Currently, the ecological environment of these areas is being improved by implementing the Three-North Shelter Forest Project. Populus simonii×P. nigra as a hybrid of P. simonii and P. nigra with the unique feature of elevated saline-alkali tolerance has been currently widely used in the Three-North Shelter Forest Project (Zhang, 2020). However, problems still exist before widely applying Populus simonii×P. nigra for environmental remediations such as low survival rate and moderate growth rate of seedlings after transplanting.
The damage of saline-alkali stress to plants is multifaceted. On the one hand, the accumulation of excess Na+ in cells can cause ion toxicity and physiological drought, leading to ABA-induced stomatal closure, limiting the entry of carbon dioxide into leaves, reducing the net photosynthetic rate and transpiration efficiency, thus limiting the normal growth of plants (Mohamed et al., 2020). At the same time, the content of reactive oxygen species can exceed the threshold, destroy the biofilm such as cell membrane and thylakoid membrane, degrade chlorophyll, and inhibit the activity of PS I and PS II (Zhang et al., 2020b). On the other hand, excessive can cause the precipitation of Ca2+ and Mg2+ by forming insoluble carbonate minerals in the soil, which reduces the bioavailability of these nutrient ions by the root system (Hui et al., 2022). Therefore, effective actions need to be taken to help plants alleviate the negative effects from saline-alkali stress.
Arbuscular mycorrhizal (AM) fungi can establish symbiotic relationships with more than 80% of terrestrial plants and are widely distributed in saline-alkali soils; they are considered as a biological tool capable to enhance plant saline-alkali tolerance (Smith and Read, 2008; Evelin et al., 2019; Wang et al., 2022b). Studies have shown that AM fungi can improve plants’ saline-alkali tolerance by promoting plant’s absorption of nutrients and water, improving rhizosphere soil conditions, improving plant photosynthesis, and inducing the expression of genes, such as Na+/H+ antiporter genes (NHX) (Smith et al., 2009; Evelin et al., 2019). For example, Kong et al. (2019) found that inoculating AM fungi in Lycopersicon esculentum Mill. under saline-alkali stress can increase chlorophyll concentration, net photosynthetic rate, stomatal conductance and transpiration rate. Yang et al. (2019a) found that inoculation with Funneliformis mosseae can increase Pyrus betulaefolia Bunge’s biomass and K+/Na+ ratio under saline-alkali stress. Although Populus is a class of plants dependent on mycorrhizal symbiosis, it takes a long time to establish a high degree of symbiotic relationship with AM fungi under normal, natural conditions (Yuan et al., 2019). Thus, inoculating the hybrid poplar species P. simonii×P. nigra with AM fungi may highly be possible to improve its saline-alkali tolerance. In addition, most current research specifically focused on the effects of AM fungi on plants under salt stress, less attention has been paid to the effects of AM fungi on plants under saline-alkali stress. Thus, detailed investigation of the role of AM fungi on populus under saline-alkali stressed environment is needed.
In order to reduce Na+ concentration in the cytoplasm, which is elevated by saline-alkali stress, plants have evolved the NHX gene family. A total of 8 NHXs have been found in Arabidopsis thaliana (Meng and Wu, 2018). AtNHX1-4 proteins localize to the tonoplast while AtNHX5 and AtNHX6 proteins localize to the Golgi and trans-Golgi networks. The six AtNHXs proteins play an important role in the transport Na+ from the cytoplasm to the vacuole and the regulation of pH and K+ concentration. The SOS1/AtNHX7 protein localized to the plasma membrane confers saline-alkali tolerance to plants through the SOS (salt overly sensitive) pathway, which excretes Na+ from cells. While SOS1B/AtNHX8 was speculated to mediate Li+ tolerance in A. thaliana, Santander et al. (2021) found that inoculation with F. mosseae and Claroideoglomus lamellosum increase the expression of LsaNHX2, LsaNHX4, LsaNHX6 and LsaNHX8, thereby improving Na+ tolerance in Lactuca sativa. However, the effects of AM fungi on host genes are different under different conditions so that more research is needed.
Therefore, in order to explore the effect of AM fungi on the saline-alkali tolerance of P. simonii×P. nigra, this pot simulation experiment was carried out. In this study, by measuring the biomass, photosynthesis, gas exchange, ion concentration, the expression of PxNHXs and other parameters of mycorrhized poplar growing in a saline-alkali environment, the mechanism of action of AM fungi on P. simonii×P. nigra’s saline-alkali tolerance was preliminarily clarified. Combined with previous studies, we made the following hypothesis: (1) inoculation with AM fungi improved the saline-alkali tolerance of P. simonii×P. nigra; (2) inoculation with AM fungi changed the expression pattern of NHX gene family in P. simonii×P. nigra, directly or indirectly affected the photosynthesis, ion absorption and other life activities of P. simonii×P. nigra, thus enhancing the saline-alkali tolerance of P. simonii×P. nigra.
2 Materials and methods
2.1 Plant material
Populus simonii×P. nigra cuttings were purchased from Lindian County, Daqing City, Heilongjiang Province, China. The cuttings were propagated by the plant factory of Northwest A&F University to obtain clone seedlings.
2.2 Fungal material
Funneliformis mosseae (BGC XJ01A) was purchased from the Institute of Plant Nutrition, Resources and Environment, Beijing Academy of Agriculture and Forestry Sciences. Propagation of F. mosseae using tobacco plants as a host. The tested inoculants included spores (the spore density was approximately 81 per gram of inoculant), mycelia and root fragments.
2.3 Growth substrate
Surface soil (5-20 cm) was obtained from the garden of Northwest Agriculture and Forestry University. The soil was passed through a 2 mm sieve and sterilized by moist heat at 121°C for 2 h twice. The river sand was passed through a 2 mm sieve, then washed, and sterilized by dry heat at 170°C for 12 h. The sterile soil and sterile sand were mixed uniformly at the 1:2 (V/V) ratio. The physicochemical properties of the soil substrate were pH 7.6 (soil and water ratio was 1:5), available N 25.77 mg·kg-1, available P 12.30 mg·kg-1, available K+ 112.67 mg·kg-1, Ca2+ 78.51 mg·kg-1, water soluble Na+ 203.54 mg·kg-1.
2.4 Experimental design
The experiment consisted of a randomized complete block design with two factors: inoculation with F. mosseae (AM) and no inoculation with F. mosseae (NM); saline-alkali stress (S) and no saline-alkali stress (N). There were 18 replicates for each treatment with a total of 72 pots.
100 mmol·L-1 Na+, pH 9.5 saline-alkali stress solution (the stress concentration was selected based on the data of the seven-year-old P. simonii×P. nigra rhizosphere soil sample and the data of the pre-test of potted plants in the greenhouse) was made by mixing 50 mmol·L-1 Na2CO3 solution and 100 mmol·L-1 NaHCO3 solution according to the volume ratio of 4:5. Each pot (7 cm × 7 cm × 5 cm) was preloaded with 230 g growth substrate. The growth substrate in 36 pots (group S) was pretreated: each pot was filled with 35 mL of saline-alkali stress solution (the maximum water content of 250 g soil matrix was about 70 mL), then after drying at 25°C, 35 mL of saline-alkali stress solution was added again. The control group without saline-alkali stress (group N) was treated by the same amount of distilled water using the same method.
Subsequently, 18 pots of each of the two groups (S and N) were selected and added with 20 g F. mosseae inoculum. The control group was added with 20 g of F. mosseae inoculum after moist heat sterilization.
One seedling was planted in one pot. Plants were cultivated in the greenhouse of the Forestry College of Northwest A&F University. The greenhouse’ room temperature was 25-28°C, light intensity was 3000 lux, photoperiod was 16/8 h, and humidity was 50%-70%. Each pot was weighed and rehydrated daily to keep the soil well moist. Each Pot was watered weekly with 25 mL of Hogland’s nutrient solution (Johnson et al., 1957) containing 1/10 Pi. After 8 weeks of culture, relevant data were measured and samples were collected.
2.5 Determination of chlorophyll fluorescence parameters and gas exchange parameters
According to the method of Wu et al. (2016), the third fully expanded leaf was selected for 30 min shading treatment. Then PAM Fluorometer (Mini-imaging-PAM, Walz, Germany) was used to measure the initial fluorescence value (Fo) and maximum fluorescence value (Fm) of leaves under saturation pulse; the minimum fluorescence value (Fo’), maximum fluorescence value (Fm’), and steady-state fluorescence value (Fs) after light adaptation. The maximum quantum yield of PSII (Fv/Fm, Fv/Fm = (Fm – Fo)/Fm), photosynthetic electron transport rate (ETR, ETR = (Fm’ – Fs)/Fm’ × Fm), non-photochemical quenching (qN, qN = (Fm − Fm’)/Fm’), photochemical quenching (qP, qP = (Fm’ – Fs)/(Fm’ − Fo’)), non-photochemical quenching coefficient according to the following formulas (NPQ, NPQ = (Fm – Fm’)/(Fm’)) and PSII actual photon quantum efficiency (ФPSII, ФPSII = (Fm’ – Fs)/Fm’) were calculated by the instrument.
According to the method of Huang et al. (2010), during the period from 8:30 am to 11:30 am, the third fully expanded leaf of each plant was selected for measuring net photosynthetic rate (Pn), stomatal conductance (gs), intercellular CO2 concentration (Ci), and transpiration rate (E) by using li-6400 portable open flow gas-exchange system (Li-Cor Inc., Lincoln, NE, United States). The measurement conditions: photo-synthetically active irradiation, 1000 µmol·m-2·s-1; temperature, 25°C; relative humidity, 60%; and CO2 concentration, 400 µmol·mol-1.
2.6 Plant height, fresh weight measurement and sample collection
After 8 weeks of culturing in the greenhouse, the height of each plant was measured using a ruler (cm). Plant samples were collected in two groups, the aboveground part and the underground part. Each 6 plants were pooled into one replicate, with three replicates per treatment. The fresh weight of the aboveground and underground parts were weighed by using an electronic analytical balance (FA2004A, Shanghai Jingda). The aboveground samples were divided into two parts with one of which immediately frozen in liquid nitrogen, then ground into powder and stored in a -80°C refrigerator and the other of which dried at 75°C to a constant weight. The underground part was divided into three parts, two of which followed the same procedure as aboveground samples and the third part was deposited in the FAA fixative to determine F. mosseae infection.
2.7 Tissue water content and relative water content of aboveground part determination
Tissue water content (TWC) and relative water content (RWC) in aboveground parts were determined according to the method of Kovar et al. (2019). We obtained the same part of the aboveground part from each sample, and weighed the fresh weight (FW). The samples were immersed in sterile distilled water for 48 hours, and the saturated fresh weight (WT) was weighed. Then the samples were dried at 75°C until constant weight, and the dry weight (DW) was weighed. Finally, the TWC ((FW – DW)/FW × 100%) and RWC ((FW – DF)/(WT – DW) × 100%) were calculated.
2.8 AM fungal infection rate detection
Roots were stained with trypan blue (Phillips and Hayman, 1970), and then mycorrhizal colonization was determined using the gridline intersection method (Giovannetti and Mosse, 1980).
2.9 Growth substrate pH determination
Referring to the method of Wang et al. (2021), weighed 10 g of air-dried soil samples that have passed through a 1 mm sieve, and measure the soil pH according to the water-soil ratio of 5:1 (W/V).
2.10 Plant Na+, K+, Ca2+ determination
After drying, the aboveground and underground samples were ground into powder, dried at 80°C to constant weight, and passed through a 20 μm sieve (Wu et al., 2016). Weighed 0.05 g of plant samples, with reference to the method of Zhou (2022), determine the concentration of Na+ and K+ in plants. Weighed 0.05 g of plant sample, with reference to the method of Porcel et al. (2016), determine the concentration of Ca2+ in plants.
2.11 Analysis of the NHX gene families of P. simonii×P. nigra
P. simonii×P. nigra genome data was obtained from http://www.wangsui.net.cn/resource/temp/Pxiaohei_DH_1_21_genome_v4.6.tar.gz (Zhou et al., 2019). The NHX protein sequences of Arabidopsis thaliana (https://phytozome-next.jgi.doe.gov/info/Athaliana_TAIR10) were used as the input sequences for the BLAST software (2.12.0+) to search for P. simonii×P. nigra NHX genes (PxNHXs). The protein sequences of candidate gene were input into HMMER software (3.3.2) and aligned with the PFAM database (https://ftp.ebi.ac.uk/pub/databases/Pfam/releases/Pfam35.0/Pfam-A.hmm.gz) to further verify their domains.
Protein physicochemical properties of PxNHXs were analyzed using ProtParam (https://web.expasy.org/protparam/). Subcellular localization predictions of PxNHXs were performed using Plant-mPLoc (http://www.csbio.sjtu.edu.cn/bioinf/plant-multi/#). Transmembrane domain predictions of PxNHXs were performed using TMHMM 2.0 (https://services.healthtech.dtu.dk/service.php?TMHMM-2.0). Signal peptide predictions for PxNHXs were performed using SignalP 5.0 (https://services.healthtech.dtu.dk/service.php?SignalP-5.0).
Phylogenetic tree of the NHX gene families of P. simonii×P. nigra, A. thaliana, Zea mays (Zorb et al., 2005), and P. trichocarpa (Tian et al., 2017) were constructed using MEGA X using the adjacency method (NJ) and 1000 bootstrap-up replicates. The phylogenetic tree was visualized by iTOL (https://itol.embl.de/).
2.12 Quantitative analysis of PxNHXs
Total RNA was extracted from these samples using the E.Z.N.A Plant RNA Kit R6827-01 (Omega Bio-Tek, Norcross, GA, USA) following the manufactory instructions strictly. RNA was reversely transcribed to cDNA using the FastQuant RT Kit KR106 (TIANGEN, Beijing, China) according to the manufactory instructions. By using NCBI Primer-BLAST (https://www.ncbi.nlm.nih.gov/tools/primer-blast/index.cgi?LINK_LOC=BlastHome), the PxNHXs’ quantitative primers was designed (Supplementary Table 1). Two housekeeping genes (NCBI accession: BU875027, GQ253565.1) were served as the reference genes (Dong et al., 2021). The qRT-PCR reaction was conducted by the CF96X real-time PCR system (Bio-Rad, Hercules, CA, USA). Each reaction mixture was 10 µL containing 1 µL diluted cDNA template, 0.5 µL forward and reverse primers (10 µmol·L-1), 5 µL ChamQ SYBR qPCR Master Mix (Vazyme, Nanjing, China), and 3 µL sterilized ddH2O. The two-step qRT-PCR was run as follows: 30 s denaturation at 95°C, 40 cycles of denaturation at 95°C for 10 s, annealing at the annealing temperature for 10 s, extension at 72°C for 20 s, followed by heating from 65 to 95°C at a rate of 0.5°C every 5 s. All samples were amplified in duplicates from the same RNA preparation and the mean value was calculated. Meanwhile, we averaged three amplified samples from each RNA and the relative expression of each target gene was calculated according to the 2−ΔΔCT protocol (Livak and Schmittgen, 2001).
2.13 Statistical analysis
All experiments were repeated at least three times. All results were expressed as the mean ± standard error (SE) in tables and figures. One-way and two-way analysis of variance (ANOVA) and Tukey’s tests using SPSS software (Version 26.0, SPSS Inc., Chicago, IL, USA) were used to evaluate significant differences across all parameters.
3 Results
3.1 Effects of saline-alkali stress on AM fungal infection rate
After 8 weeks, the roots of P. simonii×P. nigra established a symbiotic relationship with F. mosseae (Figure 1A). Under saline-alkali stress, the infection rate was significantly decreased by 56.56% (Figure 1B), which indicated that the infection activity of AM fungi was inhibited by saline-alkali stress.
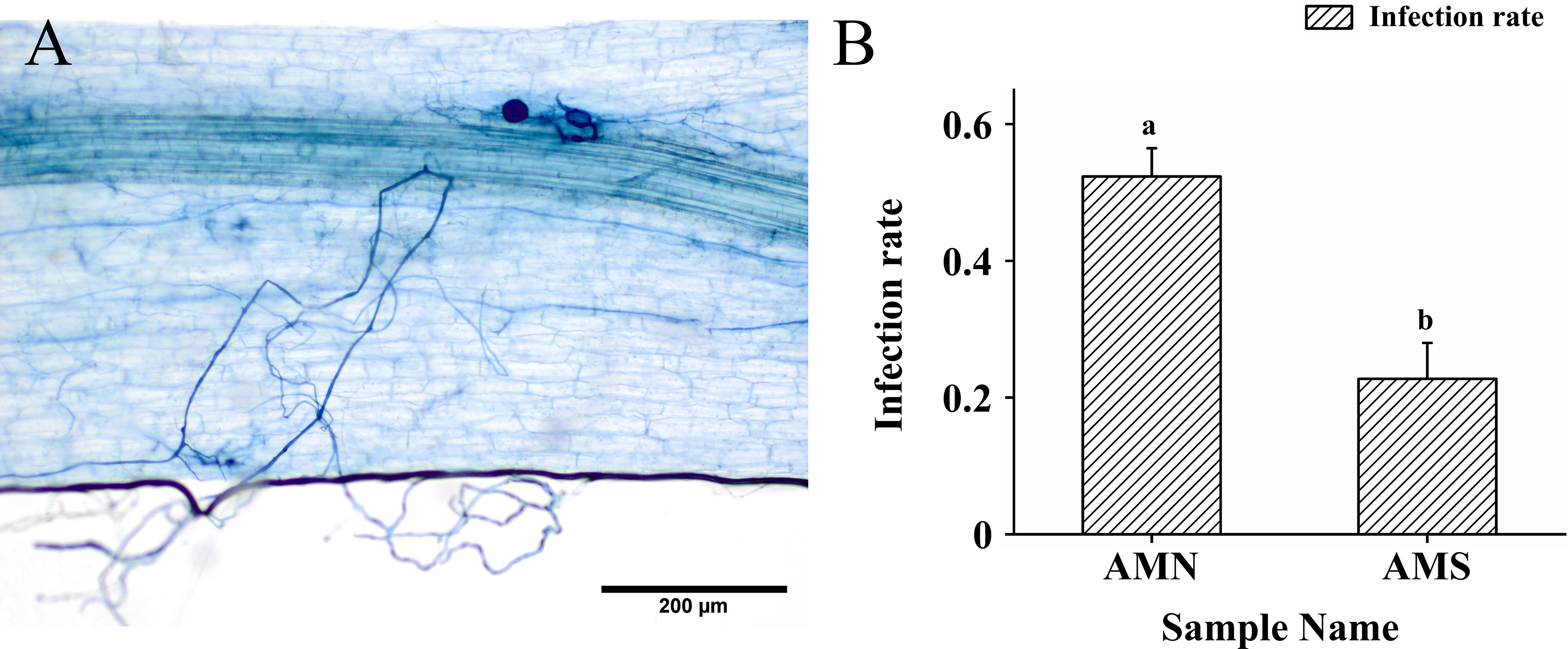
Figure 1 AM fungal infection photos and changes of AM fungal infection rate under saline-alkali stress. (A) AM fungal infection photos; (B) Changes of AM fungal infection rate under saline-alkali stress. AMN means inoculation with F. mosseae without saline-alkali stress; AMS means inoculation with F. mosseae and application of saline-alkali stress. Bars indicate mean ± standard error (n = 3). Different lowercase letters indicate significant differences between the means by Tukey’s test (P < 0.05).
3.2 Effects of AM fungal inoculation and saline-alkali stress on soil pH
As shown in Figure 2, saline-alkali treatment significantly increased soil pH while F. mosseae inoculation decreased soil pH. The soil pH of NMS (i.e., 8.54) was the highest. While the soil pH of AMS was significantly reduced by 2.93%. Two-way ANOVA showed that soil pH was significantly affected by F. mosseae inoculation and saline-alkali stress. The results indicated that AM fungi played an important role in reducing the pH of the rhizosphere soil of P. simonii×P. nigra.
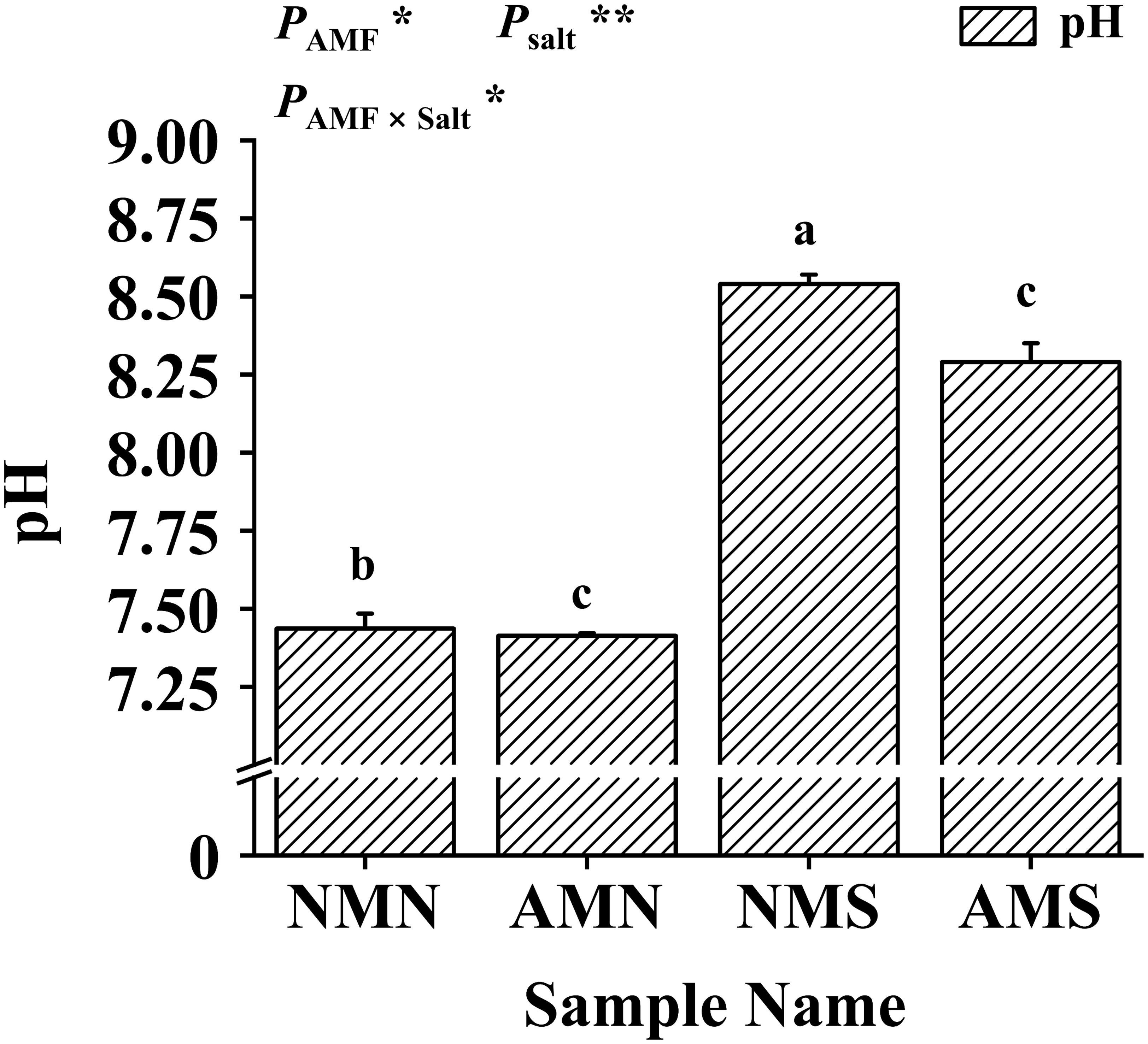
Figure 2 Effects of AM fungal inoculation and saline-alkali stress on soil pH. NMN means no F. mosseae inoculation and no saline-alkali stress; AMN means inoculation with F. mosseae without saline-alkali stress; NMS means application of saline-alkali stress without F. mosseae inoculation; AMS means inoculation with F. mosseae and application of saline-alkali stress. Bars indicate mean ± standard error (n = 3). Different lowercase letters indicate significant differences between the means by Tukey’s test (P < 0.05). Significant effect of two-way ANOVA analysis: “*” indicates P < 0.05, “**” indicates P < 0.01.
3.3 Effects of AM fungal inoculation and saline-alkali stress on plant height and fresh weight of P. simonii×P. nigra
As shown in Figure 3, saline-alkali stress significantly inhibited the growth of P. simonii×P. nigra. Compared with NMN, the plant height, fresh weight of aboveground part and fresh weight of underground part of NMS decreased by 75.25%, 53.69% and 53.69%, respectively, and AMS of which decreased by 61.16%, 49.80% and 58.52%, respectively compared with AMN. However, under the influence of F. mosseae inoculation, the plant height, fresh weight of aboveground part and fresh weight of underground part of AMS were 300.51%, 368.74% and 5.31% higher than those of NMS, respectively. Two-way ANOVA showed that the plant height and fresh weight of the aboveground part of P. simonii×P. nigra were significantly affected by AM fungal inoculation and saline-alkali treatment, indicating that AM fungi played an important role in promoting the growth of the aboveground part of P. simonii×P. nigra.
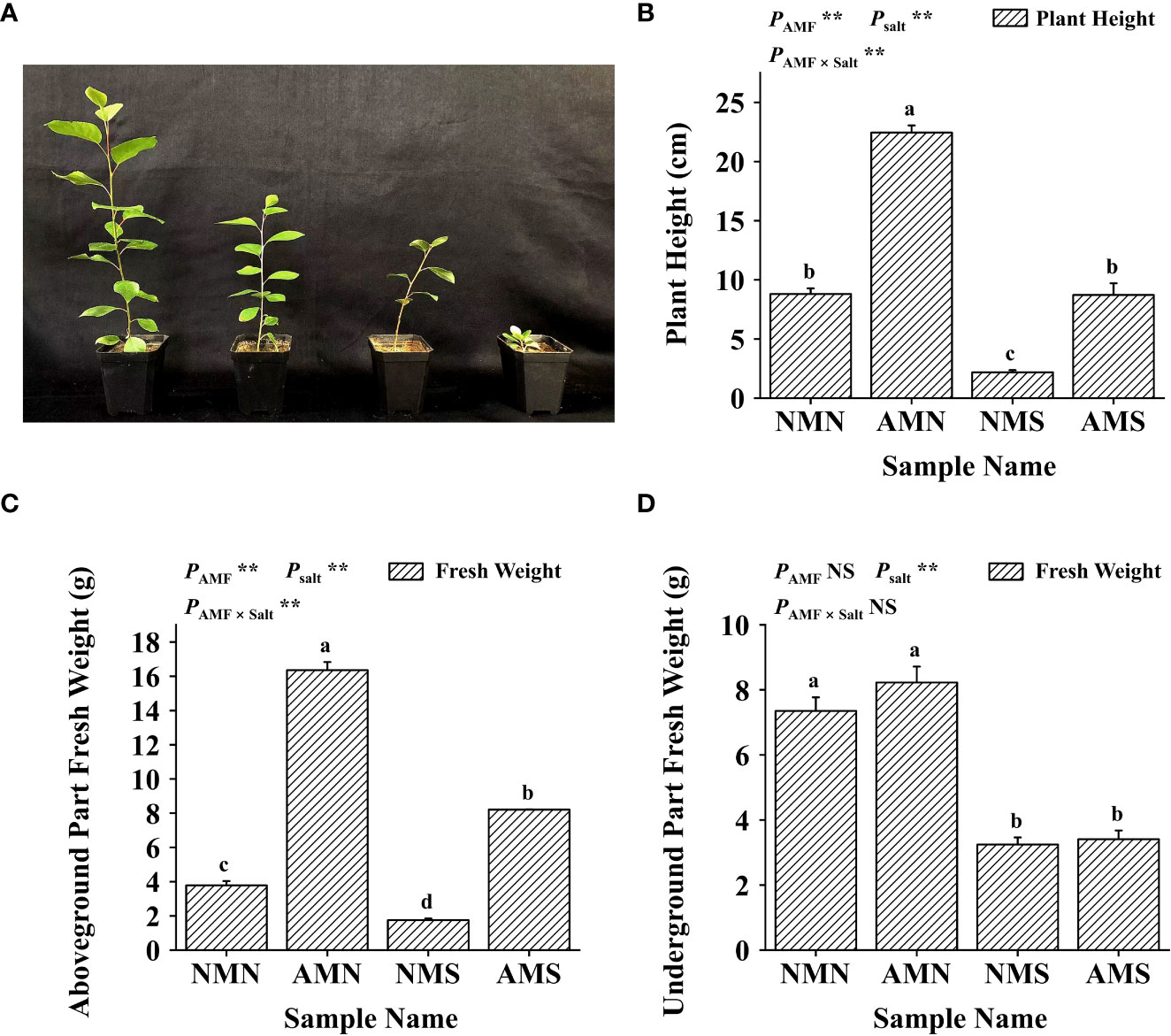
Figure 3 Effects of AM fungal inoculation and saline-alkali stress on plant height and fresh weight of P. simonii×P. nigra. (A) Photos of P. simonii×P. nigra growth status after saline-alkali stress and AM fungus inoculation for 8 weeks; from left to right, AMN, AMS, NMN and NMS. (B) Plant height histogram of P. simonii×P. nigra, bars indicate mean ± standard error (n = 18). (C) The fresh weight histogram of the aboveground part of P. simonii×P. nigra, bars indicate mean ± standard error (n = 3). (D) The fresh weight histogram of the underground part of P. simonii×P. nigra, bars indicate mean ± standard error (n = 3). NMN means no F. mosseae inoculation and no saline-alkali stress; AMN means inoculation with F. mosseae without saline-alkali stress; NMS means application of saline-alkali stress without F. mosseae inoculation; AMS means inoculation with F. mosseae and application of saline-alkali stress. Different lowercase letters indicate significant differences between the means by Tukey’s test (P < 0.05). Significant effect of two-way ANOVA analysis: “**” indicates P < 0.01; “NS” indicates no interaction (P ≥ 0.05).
3.4 Effects of AM fungal inoculation and saline-alkali stress on water content
The fresh weight of plants was affected by water content, so the tissue water content (TWC) and relative water content (RWC) of the aboveground part was further investigated. As shown in Table 1, under the influence of saline-alkali stress, the TWC of AMS and NMS was significantly increased, but RWC was not significantly affected, probably because P. simonii×P. nigra had adapted to the long-term stressed environment. Inoculation with F. mosseae could significantly increase the TWC and RWC of the aboveground parts. Compared with NMS, the TWC of AMS was significantly increased by 23.74%, and the RWC was increased by 11.70%, indicating that AM fungi play an important role in promoting plant water uptake.

Table 1 Tissue water content and relative water content of aboveground part of P. simonii×P. nigra under different treatments.
3.5 Effects of AM fungal inoculation and saline-alkali stress on gas exchange parameters
Plants synthesize organic matter through photosynthesis for maintaining a symbiotic relationship with mycorrhizae and for their own growth. As shown in Figure 4, F. mosseae inoculation increased the Pn (net photosynthetic rate, 140.57%), gs (stomatal conductance, 183.33%), Ci (intercellular CO2 concentration, 6.88%) and E (transpiration rate, 173.11%) of P. simonii×P. nigra without salt-alkali stress. The application of saline-alkali stress resulted in a decrease in the Pn of NMS (40.25%) and a significant increase in the Ci (15.97%). For AMS, although saline-alkali stress led to a significant decrease in Pn (29.28%), gs (45.27%), Ci (11.05%) and E (29.69%) (compared with AMN), the Pn (70.13%), gs (55.07%) and E (92.03%) were still higher than those of NMN. Two-way ANOVA analysis showed that gs and Ci were affected between saline-alkali stress and AM fungi interaction, indicating that AM fungi may promote the growth of P. simonii×P. nigra by regulating these two pathways under saline-alkali stress.
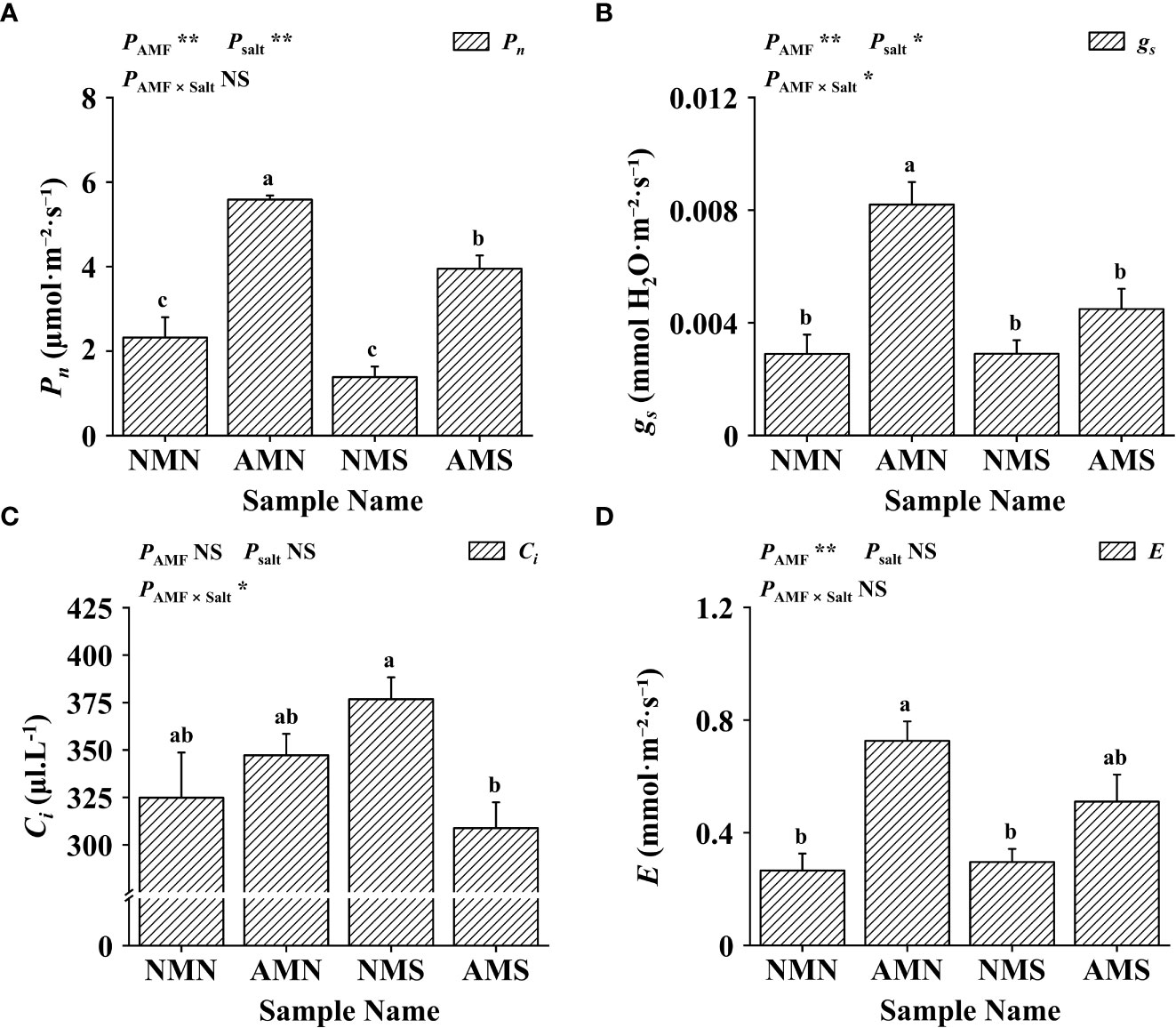
Figure 4 Effects of AM fungal inoculation and saline-alkali stress on gas exchange parameters. (A) Effects of AM fungal inoculation and saline-alkali stress on Pn value; (B) Effects of AM fungal inoculation and saline-alkali stress on gs value; (C) Effects of AM fungal inoculation and saline-alkali stress on Ci value; (D) Effects of AM fungal inoculation and saline-alkali stress on E value. NMN means no F. mosseae inoculation and no saline-alkali stress; AMN means inoculation with F. mosseae without saline-alkali stress; NMS means application of saline-alkali stress without F. mosseae inoculation; AMS means inoculation with F. mosseae and application of saline-alkali stress. Pn means net photosynthetic rate, gs means stomatal conductance, Ci means intercellular CO2 concentration, and E means transpiration rate. Bars indicate mean ± standard error (n = 5). Different lowercase letters indicate significant differences between the means by Tukey’s test (P < 0.05). Significant effect of two-way ANOVA analysis: “*” indicates P < 0.05, “**” indicates P < 0.01; “NS” indicates no interaction (P ≥ 0.05).
3.6 Effects of AM fungal inoculation and saline-alkali stress on chlorophyll fluorescence
As shown in Figure 5, the effects of saline-alkali stress and AM fungal inoculation on the chlorophyll fluorescence parameters of P. simonii×P. nigra were different. Saline-alkali stress significantly reduced the Fv/Fm value regardless of whether F. mosseae was inoculated or not. However, the Fv/Fm value of AMS was still significantly higher than that of NMS (3.10%), which was similar to that of NMN. For NPQ, ФPSII, qN, qP and ETR, the inoculation with F. mosseae under saline-alkali stress had opposite effects on these parameters. Compared with NMN, NPQ and qN of NMS decreased by 19.90% and 2.39%, respectively while ФPSII, qP and ETR increased by 13.28%, 9.82% and 13.61%, respectively. Although inoculation with F. mosseae increased the values of ФPSII, qP and ETR (compared with NMN), under the influence of saline-alkali stress, compared with AMN, the ФPSII, qP and ETR values of AMS decreased by 16.63%, 2.61% and 15.50%, respectively, and at the same time, NPQ and qN increased by 23.67% and 13.64%, respectively. However, the ФPSII (11.08%), qP (0.84%) and ETR (10.64%) of AMS were still higher than those of NMS. Two-way ANOVA analysis showed that AM fungal inoculation and saline-alkali stress significantly affected NPQ, ФPSII, qN and ETR.
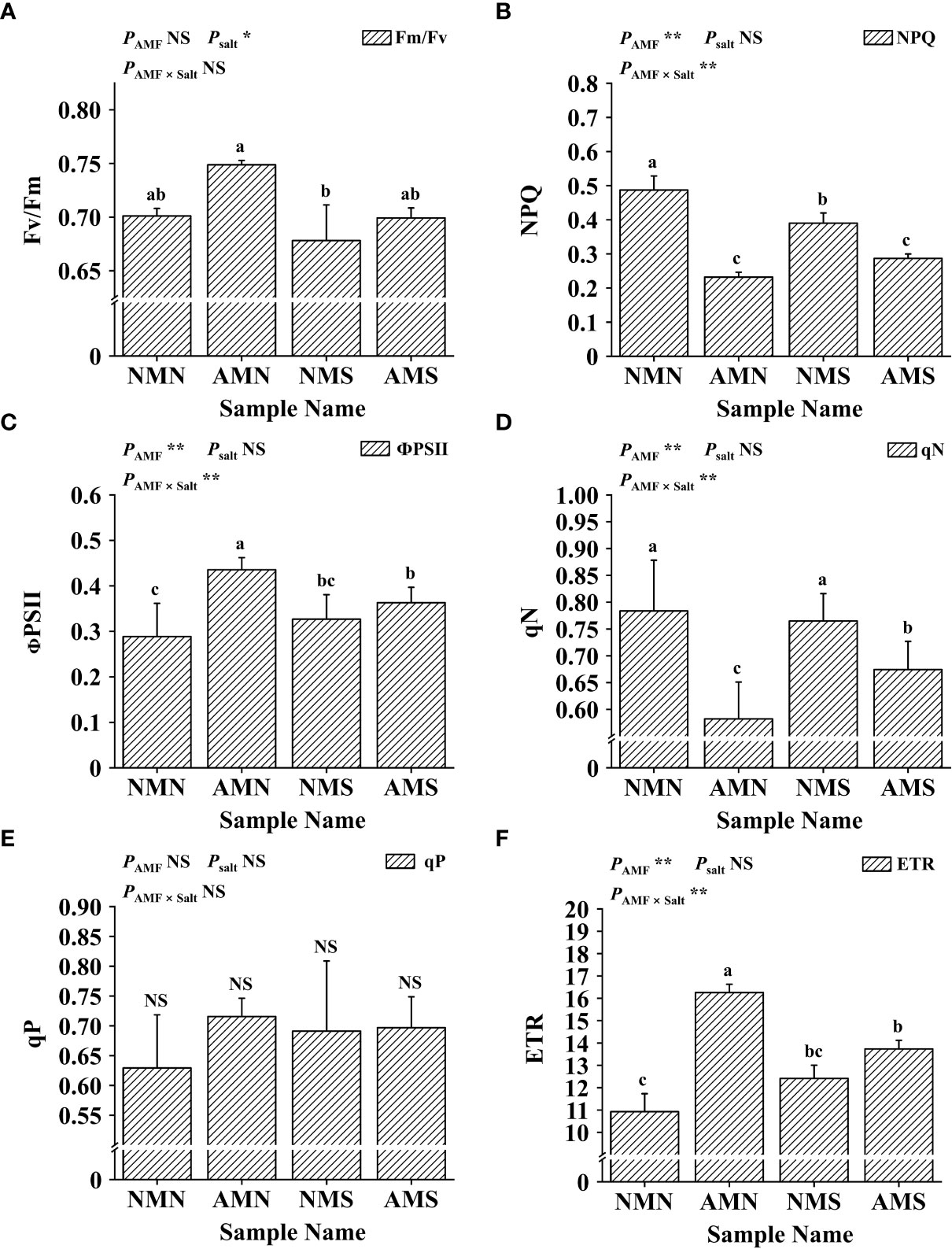
Figure 5 Effects of AM fungal inoculation and saline-alkali stress on chlorophyll fluorescence. (A) Effects of AM fungal inoculation and saline-alkali stress on Fv/Fm value; (B) Effects of AM fungal inoculation and saline-alkali stress on NPQ value; (C) Effects of AM fungal inoculation and saline-alkali stress on ФPSII value; (D) Effects of AM fungal inoculation and saline-alkali stress on qN value; (E) Effects of AM fungal inoculation and saline-alkali stress on qP value; (F) Effects of AM fungal inoculation and saline-alkali stress on ETR value. Fv/Fm means maximal photosystem II quantum yield; NPQ means non-photochemical quenching; ФPSII means the efficiency of photosystem II; qN means non-photochemical quenching coefficient; qP means photochemical quenching coefficient; ETR means relative electron transfer rate. NMN means no F. mosseae inoculation and no saline-alkali stress; AMN means inoculation with F. mosseae without saline-alkali stress; NMS means application of saline-alkali stress without F. mosseae inoculation; AMS means inoculation with F. mosseae and application of saline-alkali stress. Bars indicate mean ± standard error (n = 5). Different lowercase letters indicate significant differences between the means by Tukey’s test (P < 0.05). Significant effect of two-way ANOVA analysis: “*” indicates P < 0.05, “**” indicates P < 0.01; “NS” indicates no interaction (P ≥ 0.05).
3.7 Effects of AM fungus inoculation and saline-alkali stress on Na+, K+ and Ca2+ concentrations of P. simonii×P. nigra
As shown in Table 2, the saline-alkali treatment caused an increase in the Na+, K+ and Ca2+ concentrations of P. simonii×P. nigra, while the Na+, K+ and Ca2+ concentrations were further increased after inoculation with AM fungi. Compared with NMS, the Na+, K+ and Ca2+ concentrations in the aboveground part of AMS were increased by 444.92%, 63.14% and 62.31%, respectively while those of the underground part were increased by 106.28%, 8.53% and 0.93%, respectively. Two-way ANOVA showed that the Na+, K+ and Ca2+ concentrations in the aboveground and underground parts of P. simonii×P. nigra were significantly affected by AM fungal inoculation and saline-alkali treatment. It suggested that AM fungal inoculation in saline-alkali environment played an important role in promoting plant element uptake.
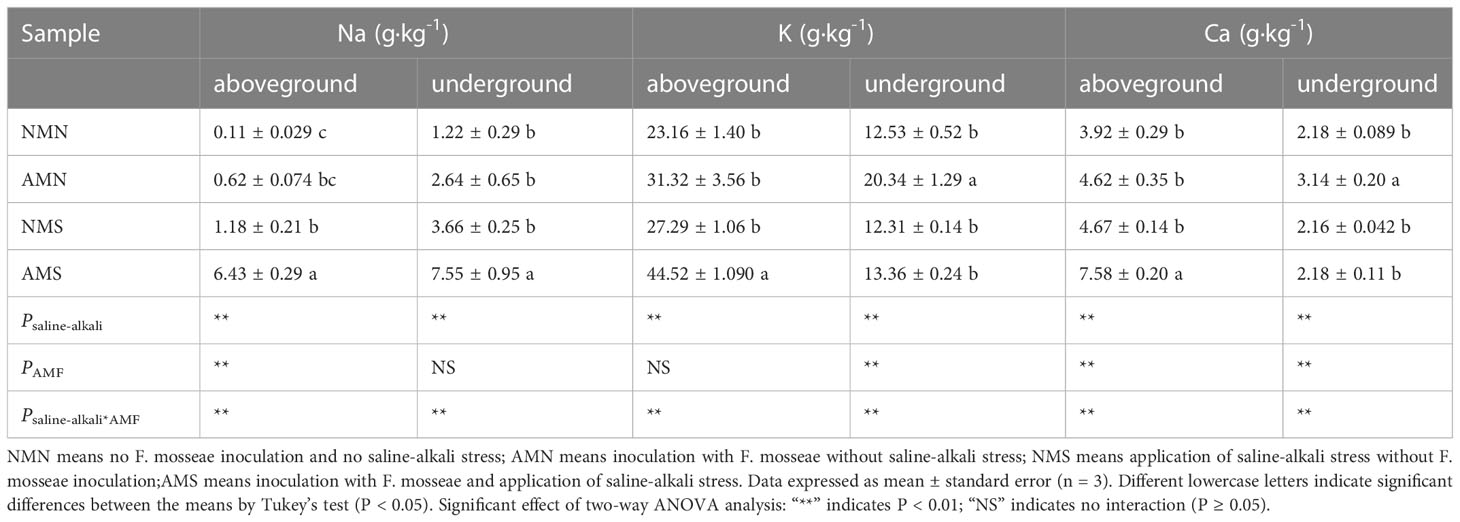
Table 2 Na+, K+ and Ca2+ concentrations in aboveground and underground parts of P. simonii×P. nigra.
3.8 Identification and analysis of NHX gene families of P. simonii×P. nigra
A total of 8 NHX gene family members were identified in the P. simonii×P. nigra genome (Supplementary Table 2). The protein length of the eight PxNHXs ranged from 318 (Px_DH25939) to 1147 (Px_DH21638) amino acids; the molecular weight of the protein ranged from 35.13 kDa (Px_DH25939) to 127.02kDa (Px_DH21638); the isoelectric point ranged from 5.48 (Px_DH25989) to 8.85 (Px_DH28184); the number of transmembrane domains ranged from 7 (Px_DH25939) to 12 (Px_DH18325 and Px_DH21638). Subcellular localization showed that six PxNHXs were localized to vacuole and other two PxNHXs were localized to the cell membrane.
To further clarify the corresponding gene functions and evolutionary relationships of PxNHXs, a phylogenetic tree was constructed (Figure 6). The eight PxNHXs were divided into three clades: Px_DH25939, Px_DH28184, Px_DH21008, Px_DH25989 and Px_DH11117 were divided into the same clades, which were similar to AtNHX2 in function; Px_DH30127 was divided into one branch, which was functionally similar to AtNHX6; Px_DH21638 and Px_DH18325 were divided into the same clade and were functionally similar to AtNHX7.
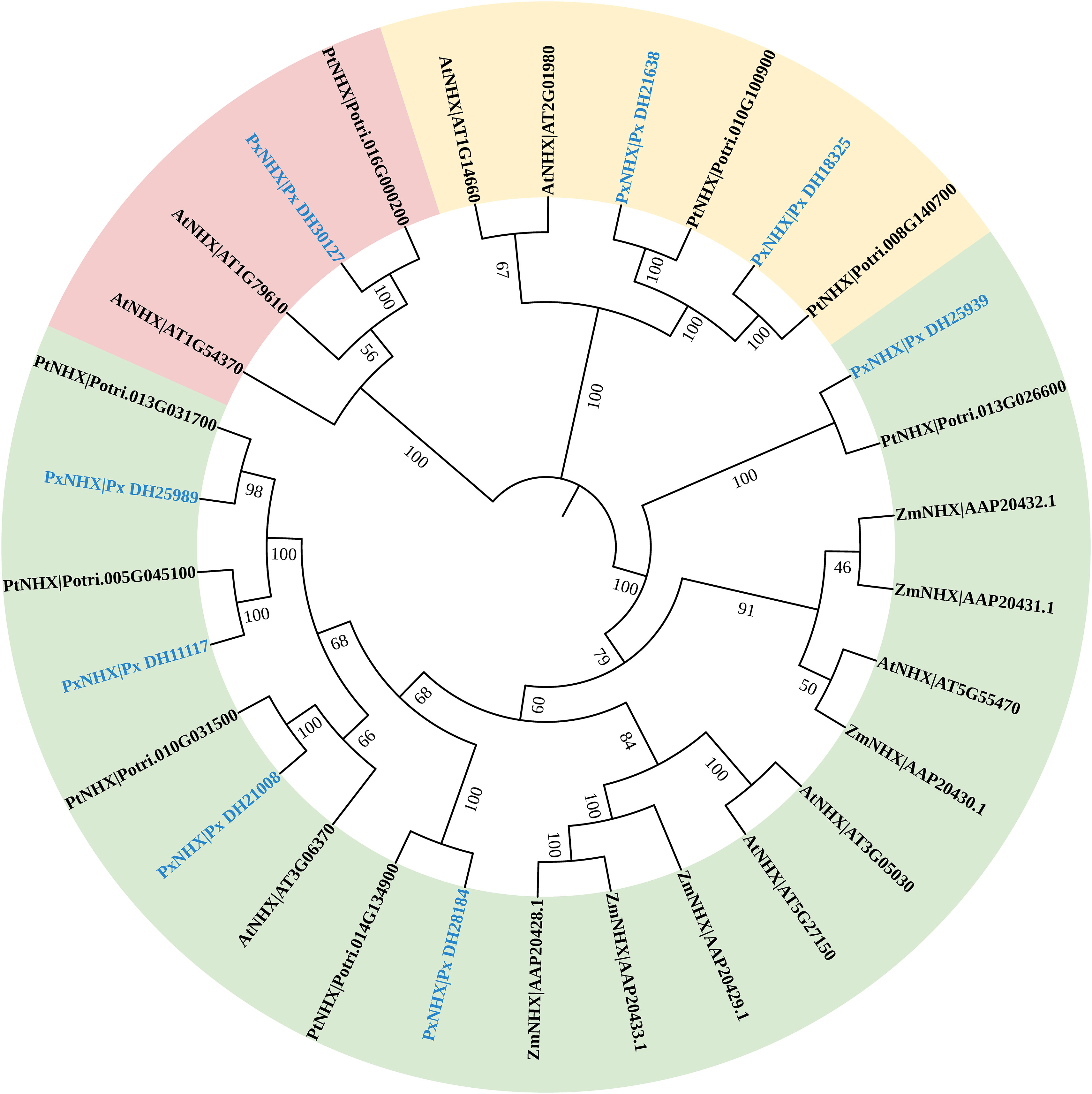
Figure 6 The evolutionary tree of the NHX gene family of P. simonii×P. nigra, Arabidopsis thaliana, P. trichocarpa and Zea mays.
3.9 Effects of AM fungal inoculation and saline-alkali stress on the expression of PxNHXs
Through qPCR analysis of NHX gene family, the effects of AM fungus inoculation on saline-alkali tolerance of P. simonii×P. nigra were explored from the microscopic level. In the aboveground part (Figure 7A), saline-alkali stress led to the down-regulated expression of Px_DH21008, Px_DH25989, Px_DH30127, Px_DH21638, and Px_DH18325 in NMS to varying degrees (2.45%-25.36%). Under saline-alkali stress, inoculation with F. mosseae up-regulated the expression of PxNHXs except Px_DH25939. Among them, Px_DH21008, Px_DH25989, Px_DH11117 and Px_DH21638 were significantly affected by F. mosseae inoculation, and their expressions were up-regulated. Compared with NMS, their expression levels in AMS were increased by 182.95%, 57.24%, 27.34%, and 143.56%, respectively. In the underground part (Figure 7B), PxNHXs’ expressions were more active. Under the saline-alkali stress, these genes were up-regulated by 200.99% (Px_DH21008)-1562.91% (Px_DH21638) in NMS. Inoculation with F. mosseae also induced further up-regulation of these genes. In AMS, the expression of these genes increased by 254.16% (Px_DH25939)-34762.16% (Px_DH21638) compared with NMN, indicating that AM fungi endow plants with higher saline-alkali tolerance by inducing the up-regulated expression of these genes. One-way ANOVA of the underground part showed that saline-alkali stress mainly affected the expression of Px_DH28184 and Px_DH30127. While AM fungi significantly affected the expression of PxNHXs except Px_DH25939. Two-way ANOVA indicated that the expression of Px_DH11117 and Px_DH30127 in the underground part were affected by the interaction of AM fungal inoculation and saline-alkali treatment.
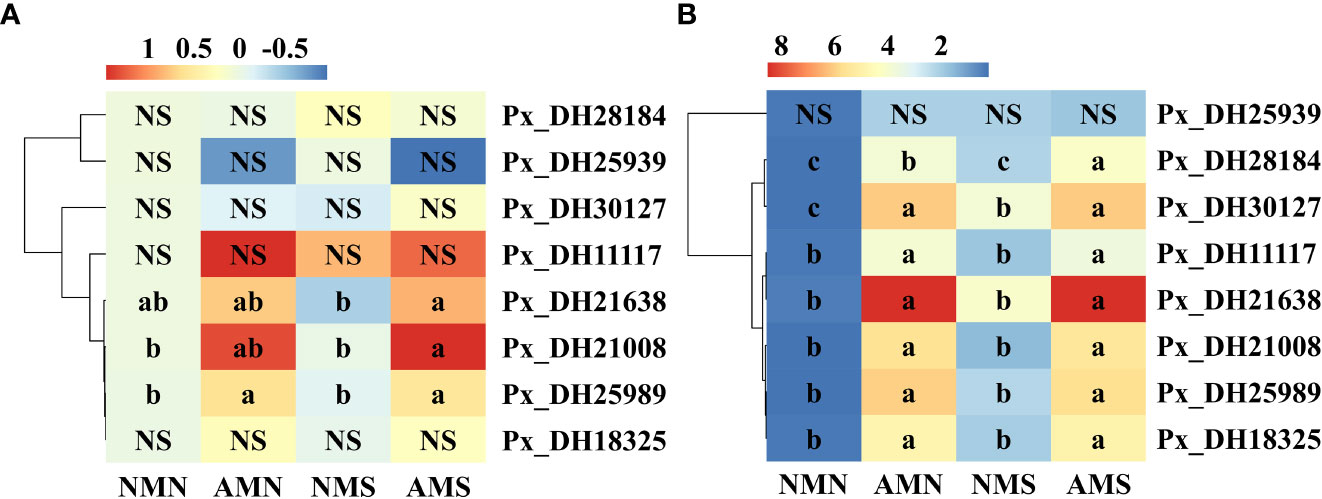
Figure 7 Expression thermogram of NHX gene family in P. simonii×P. nigra. (A) Expression thermogram of NHX gene family in aboveground part of P. simonii×P. nigra. (B) Expression thermogram of NHX gene family in underground part of P. simonii×P. nigra. NMN means no F. mosseae inoculation and no saline-alkali stress; AMN means inoculation with F. mosseae without saline-alkali stress; NMS means application of saline-alkali stress without F. mosseae inoculation;AMS means inoculation with F. mosseae and application of saline-alkali stress. The colour scale represents the log2 value of the gene expression (n = 3). From red to blue, the expression level was from high to low. Different lowercase letters indicate significant differences between the means by Tukey’s test (P < 0.05). “NS” indicates no interaction (P ≥ 0.05). The results of two-way ANOVA for each gene are presented in the Supplementary Table 3.
3.10 Correlation analysis of PxNHXs induced by AM fungi and growth state of P. simonii×P. nigra
In order to explore the potential relationship between PxNHXs induced by AM fungi and the growth status of P. simonii×P. nigra, Pearson’s correlation analysis was performed on the above parameters. In aboveground parts (Figure 8), inoculation with AM fungi was significantly correlated with the expression of Px_DH21008, Px_DH25989, Px_DH11117, Px_DH25939, Px_DH21638, Px_DH18325. The expression of these genes significantly affected the physiological activities (TWC, RWC, plant weight and FW), photosynthesis (ETR, qN, qP, NPQ and ФPSII), gas exchange (Pn、gs and E) and ion concentration (Na+, K+ and Ca2+) of the aboveground parts, suggesting that AM fungi could affect the growth status of P. simonii×P. nigra by affecting the expression of PxNHXs in the aboveground parts.
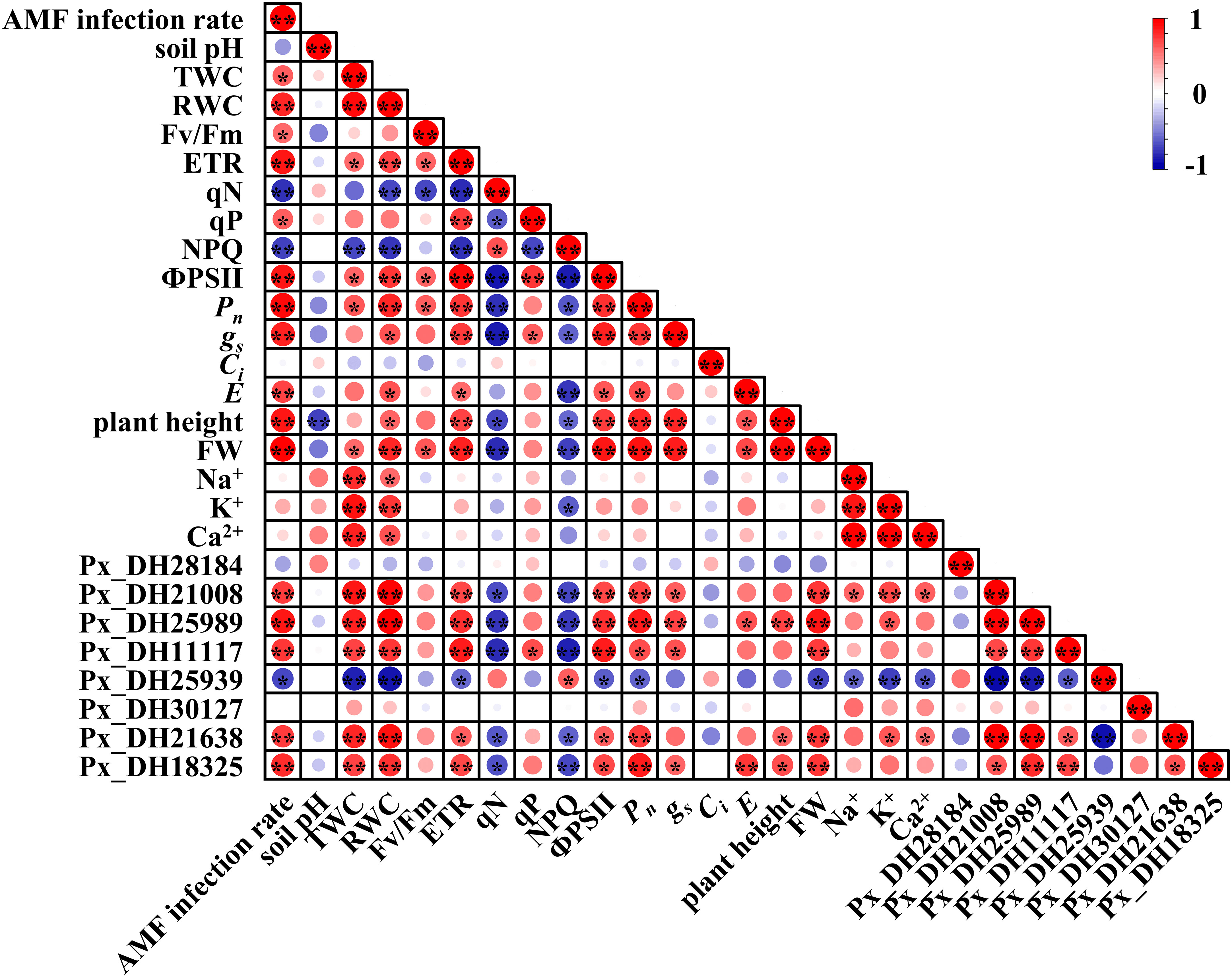
Figure 8 Thermogram of Pearson’s correlation between AM fungal infection and different parameters in aboveground part. TWC, tissue water content; RWC, relative water content; Fv/Fm, the maximum quantum yield of PSII; ETR, photosynthetic electron transport rate, qN, non-photochemical quenching, qP, photochemical quenching, NPQ, non-photochemical quenching coefficient according to the following formulas; ФPSII, PSII actual photon quantum efficiency; Pn, net photosynthetic rate; gs, stomatal conductance; Ci, intercellular CO2 concentration; E, transpiration rate; FW, fresh weight. Each circle indicates the Pearson’s correlation coefficient of a pair of parameters. “*” indicates P < 0.05, “**” indicates P < 0.01.
In underground parts (Figure 9), inoculation with AM fungi was significantly correlated with the PxNHXs excepted Px_DH25939. At the same time, these genes were also related to the Na+, K+, Ca2+ concentrations in the underground part of P. simonii×P. nigra. Although AM fungal inoculation had no direct and significant correlation with root Na+ concentration and rhizosphere soil pH, the Px_DH28184, Px_DH30127 were significantly correlated with underground Na+ concentration, while Na+ concentration was significantly correlated with underground FW and rhizosphere soil pH. This result implies that AM fungi can alleviate saline-alkali stress by regulating the expression of these two genes to regulate root growth and reduce soil pH.
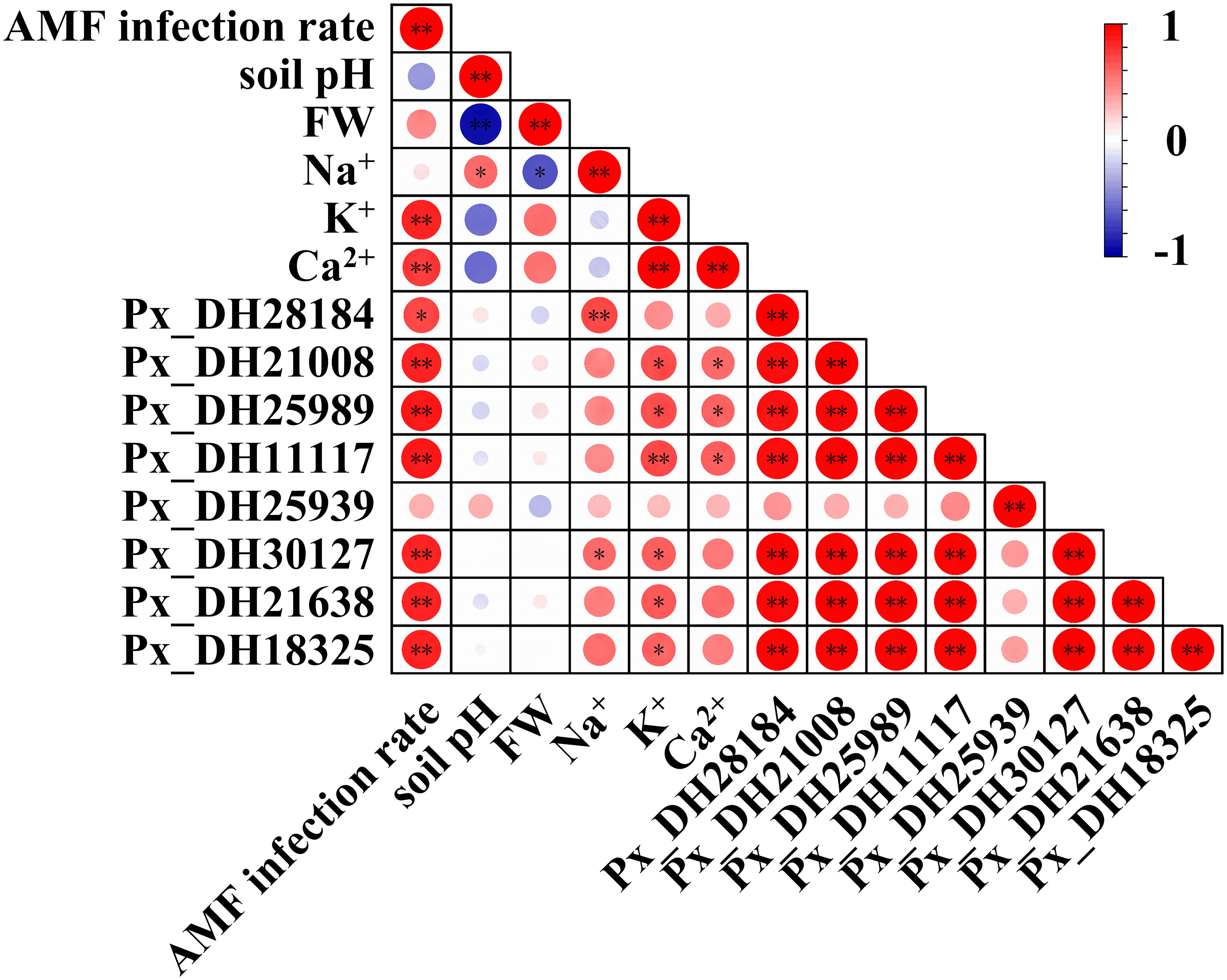
Figure 9 Thermogram of Pearson’s correlation between AM fungal infection and different parameters in underground part. FW, fresh weight. Each circle indicates the Pearson’s correlation coefficient of a pair of parameters. “*” indicates P < 0.05, “**” indicates P < 0.01.
4 Discussion
Saline-alkali stress seriously endangers the normal growth of plants (Yu et al., 2021). To mitigate this effect, plants take a variety of methods (Fang et al., 2021). Some plants can tolerate saline-alkali environments better by establishing a symbiotic relationship with AM fungi in the rhizosphere (Xun et al., 2015; Zu et al., 2018). Our results showed that the inoculation with F. mosseae improved the saline-alkali tolerance of P. simonii×P. nigra. At the same time, the effects of F. mosseae on the saline-alkali tolerance of P. simonii×P. nigra was manifold.
Under saline-alkali stress, the activities of plants and microorganisms could be inhibited (Arab et al., 2018; Ma et al., 2022a). Previous studies have shown that the saline-alkali tolerance of plants was positively correlated with biomass (Chen et al., 2017). In our study, although the infection activity of F. mosseae was inhibited by saline-alkali stress, the establishment of the symbiotic relationship between F. mosseae and P. simonii×P. nigra still promoted the increase of plant height and fresh weight of AMS aboveground parts, indicating that mycorrhizated P. simonii×P. nigra had higher saline-alkali tolerance than non-mycorrhizated P. simonii×P. nigra.
Plants absorb CO2 and water from the outside, and produce organic matter through a series of redox reactions on chloroplasts for plant growth (Khan et al., 2022). The occurrence of saline-alkali stress hinders the normal water absorption of plants and increases the concentration of Na+ and reactive oxygen species in cells. At the same time, the physiological drought of the plant is induced, and the plant reduces the evaporation of water by closing the stomata to retain more water for diluting the Na+ in the cells, thereby reducing the stomatal conductance (Athar et al., 2015). On the other hand, salt stress reduces photosynthesis by inhibiting photosystem II (PSII) activity and destroying chlorophyll pigments through Na+ accumulation. In salt-sensitive sweet sorghum, salt stress significantly reduced its Pn, ФPSII, gs, and Ci. In contrast, the salt-tolerant sweet sorghum genotypes could still maintain high photosynthetic efficiency (Sui et al., 2015). Therefore, the strength of photosynthetic ability is also one of the important indicators to measure the stress tolerance of plants. Many studies have confirmed that AM fungi can regulate the expression of photosynthesis-related genes by establishing a symbiotic relationship with plants, increasing the photosynthetic activity of host plants, regulating stomatal conductance, and promoting plant growth in stressful environments (Chandrasekaran et al., 2019; Diao et al., 2021). Under stressed conditions, AM fungi transfer the water in the soil gap to the surrounding of the root through the extra-root hyphae, and on the other hand increase the transpiration rate and stomatal conductance of the host to accelerate water absorption (Zhu et al., 2015). Our study also found that the water content, stomatal conductance and transpiration rate of AMS were significantly higher than those of NMS. This shows that after the occurrence of stress, the roots of P. simonii×P. nigra can absorb more water by relying on F. mosseae to alleviate the physiological drought in the body, so that the stomatal opening degree is higher than that of NMS, which is conducive to the progress of photosynthesis. The replenishment of water and carbon dioxide allows photosynthesis to proceed smoothly. Although the Pn of P. simonii×P. nigra in AMS and NMS decreased significantly, inoculation with F. mosseae still increased the Pn value. Therefore, the P. simonii×P. nigra in AMS can produce more organic matter for its own growth and secrete organic acids to improve the soil environment of the roots, obtain more nutrients, and form a virtuous circle. In salt-tolerant and sensitive genotypes, Fv/Fm and ΦPSII decreased with increasing NaCl concentration, and the decrease was more pronounced in plants sensitive to salt stress (Yang et al., 2019c). Due to salt-induced increase in Fo and significant decrease in Fm, the Fv/Fm and ФPSII values were significantly reduced after salt stress treatment by Wu et al. (2016), resulting in a decrease in the biomass of P. cathayana, while inoculation with Rhizophagus intraradices could increase the Fm/Fv and ФPSII values. In this study, it was also found that inoculation of F. mosseae under saline-alkali stress increased the Fv/Fm, ФPSII and ETR values of P. simonii×P. nigra. AMS showed higher Fv/Fm and ФPSII values than NMS, indicating that NMS had a more serious disorder in the ФPSII electron transport chain. Studies have shown that the value of ФPSII and ETR is positively correlated with the tolerance of plants to adversity (Jia et al., 2019). Our results further confirmed that P. simonii×P. nigra exhibited higher saline-alkali tolerance through long-term symbiosis with F. mosseae under the same stressed environment.
The increase of soil pH inhibited the growth and activity of roots, resulting in the immobilization of bioavailable nutrients and then reducing the uptake efficiency of these nutrients by plants (Robin et al., 2016; Neina, 2019). Roots can secrete organic acids to reduce soil pH, dissolve carbonate minerals and release related elements for plant’s absorption, and maintain pH stability and ion balance in cells (Fazeli-Nasab et al., 2022). However, due to factors such as weakened root vigor, plants cannot absorb enough nutrients for the synthesis of organic acids in a short period of time, so they cannot reduce soil pH to a suitable growth level in a short period of time. Inoculation with AM fungi accelerates this process, assisting the plant to reduce the pH of the rhizosphere soil in a shorter time. In Citrus reticulata, Glomus epigaeum lowered soil pH by inducing the secretion of phenolic acids from roots (Zhang et al., 2020a). The pH of the rhizosphere soil was also significantly decreased after inoculation with F. mosseae in P. simonii×P. nigra. This indicates that F. mosseae can promote the secretion of organic acids in P. simonii×P. nigra to improve the soil environment, but which organic acid secretion is affected needs further study. In addition to inducing plants to secrete organic acids, the mycelia will also secrete organic acids for mineral weathering, while absorbing metal cations in the soil and releasing H+, thereby reducing the pH of the rhizosphere soil (Luthfiana et al., 2021; Wang et al., 2022a). Our findings also support this view. The correlation analysis of the underground part of P. simonii×P. nigra showed that the pH of the soil was significantly correlated with the Na+ concentration in the underground part. Whether under saline-alkali stress or not, the presence of AM fungi increased Na+ concentration in P. simonii×P. nigra roots and decreased rhizosphere soil pH. Ma et al. (2022b) found that AM fungi mediated the uptake of Na+ and the efflux of H+ in the root of Ziziphus jujuba under salt stress. These results indicated that AM fungi could improve the rhizosphere soil environment and reduce stress damage to the root system by promoting the absorption of Na+ and releasing H+ to reduce the pH of the rhizosphere.
The increase of single Na+ concentration in cells disrupts ion homeostasis, affects enzyme activity and cell membrane stability, and thus affects normal cell growth. Since Na+ and K+ have similar structures, they compete with each other for adsorption sites and active sites, resulting in reduced K+ absorption and inhibition of K+-dependent enzymatic activities and metabolic processes (Assaha et al., 2017). At the same time, Na+ can also replace Ca2+ on the cell membrane, thereby destroying the membrane structure (Lu et al., 2018). Therefore, plants need to absorb more K+ and Ca2+ from the soil to increase the concentration of K+ and Ca2+ in the body and improve competitiveness. Studies on Lycopersicon esculentum Mill. showed that inoculation with AM fungi (Glomus clarum and G. intraradices) under saline-alkali stress promoted its uptake of K+ and Ca2+ (Kong et al., 2019). Our study also showed similar results but did not show higher K+/Na+ and Ca2+/Na+ values. The reason might be that AM fungus affected the distribution of Na+ in P. simonii×P. nigra, and a large amount of Na+ was sequestered in the vacuole through certain measures. As a result, the reduction of Na+ in the cytoplasm makes it unnecessary for plants to accumulate more K+ and Ca2+ to balance Na+.
To complete the process of Na+ sequestration in a short period of time, plants need more transporters, and this process is regulated by gene expression. The NHX gene family is responsible for the compartmentalization and efflux of Na+ in cells. Meng and Wu (2018) analyzed the NHX gene family of 72 individuals of 31 species in Populus and found that the Populus NHX family contains 8 members. By the bioinformatics analysis, 8 NHX genes were also found in P. simonii×P. nigra. Among them, 6 genes were highly similar to AtNHX2 and AtNHX6. We guess that these six genes are responsible for regulating Na+ compartmentalization in cells. A study showed that overexpressing the wheat TaNHX2 gene in Solanum melongena L. enhanced its salt tolerance (Yarra, 2019; Yarra and Kirti, 2019). Under 200 mM salt stress, the Na+ concentration in the transgenic tomato was significantly higher than that of the wild type, showing a higher Na+ capacity. At the same time, under salt stress, the growth performance, photosynthesis, transpiration rate, and stomatal conductance of the transgenic tomato were superior to those of the wild type. Inoculation with AM fungi also produced a similar response in P. simonii×P. nigra. Compared with NMS, AM fungi increased the expression levels of Px_DH28184, Px_DH30127, Px_DH11117, Px_DH21638 and Px_DH21008 in P. simonii×P. nigra shoots under saline-alkali stress. At the same time, the results of correlation analysis also showed that these genes were related to poplar fresh weight, photosynthesis, gas exchange and ion concentration, and these parameters were also improved by AM fungal inoculation. Therefore, there is such a possibility that under saline-alkali stress, AM fungi can improve the salinity-alkali tolerance of poplar, growth performance and photosynthesis by inducing the expression of PxNHXs. The SOS pathway is the first saline-alkali stress signal transduction pathway established in plants (Fang et al., 2021). Px_DH21638 and Px_DH18325 are highly similar to AtNHX7/SOS1, we guess that they are involved in the SOS pathway and are responsible for regulating Na+ efflux in cells. SpSOS1 can improve plant salt tolerance by regulating ion homeostasis and protecting plasma membrane (PM) from oxidative damage under salt stress (Zhou et al., 2018). In Pistacia vera L. cv. Ohadi, R. irregularis enhanced the host’s salt tolerance by inducing up-regulated expression of the SOS1 gene (Abbaspour et al., 2021). Both Px_DH21638 and Px_DH18325 were up-regulated in aboveground and underground parts. At the same time, these two genes were also related to parameters such as fresh weight, photosynthesis, gas exchange and ion concentration of P. simonii×P. nigra. Therefore, AM fungi may also affect the salinity tolerance of P. simonii×P. nigra by regulating the expression of these genes. Interestingly, pre-mycorrhizal treatment also induced the up-regulated expression of Px_DH21638 and Px_DH18325 even without saline-alkali stress, which further illustrates the importance of pre-mycorrhizal treatment.
5 Conclusions
In summary, inoculation with AM fungi improved the saline-alkali tolerance of P. simonii×P. nigra. This increased tolerance may be conferred by AM fungi altering the expression of the NHX gene family. This study found 8 NHX gene family members in P. simonii×P. nigra. The expression levels of Px_DH28184, Px_DH30127, Px_DH11117, Px_DH21638, Px_DH21008, Px_DH21638 and Px_DH18325 were affected by AM fungi and changed under saline-alkali stress. On the one hand, the changes in the expression of these genes increased the concentration of Na+, K+, and Ca2+ in the underground part, reduced the pH and Na+ concentration in the rhizosphere soil, and improved the rhizosphere soil environment. On the other hand, it adjusted the distribution of Na+ in the aboveground part cells and increased the tissue water content of poplar. Thus reducing the toxic effect of Na+ on chloroplasts, increasing stomatal conductance, transpiration rate and net photosynthetic rate, stabilizing light and electron transport chains, and improving photosynthesis. Through these methods, the rapid growth of mycorrhizal P. simonii×P. nigra in the saline-alkali stress environment was finally realized. However, it is not enough to only study the changes within a plant at a single time, and the gene expression in plants changes dynamically. In the future, it is necessary to focus on the influence of mycorrhizae on the expression of the NHX gene family in P. simonii×P. nigra during the initial period of saline-alkali stress. Our findings contribute to a deep understanding of the mechanism of AMF-induced saline-alkali tolerance and lay the foundation for further improvement of plant saline-alkali tolerance.
Data availability statement
The datasets presented in this study can be found in online repositories. The names of the repository/repositories and accession number(s) can be found in the article/Supplementary Material.
Author contributions
Conceptualization, FD. Methodology and investigation, FD, YW and JT. Data curation, FD. Writing—original draft preparation, FD and TX. Writing—review and editing, TX. Supervision and project administration, MT. Funding acquisition, MT. All authors contributed to the article and approved the submitted version.
Funding
This research was funded by the Laboratory of Lingnan Modern Agriculture Project (NZ2021025), the National Natural Science Foundation of China (32071639), and the National Key Research and Development Program of China (2018YFD0600203-3).
Conflict of interest
The authors declare that the research was conducted in the absence of any commercial or financial relationships that could be construed as a potential conflict of interest.
Publisher’s note
All claims expressed in this article are solely those of the authors and do not necessarily represent those of their affiliated organizations, or those of the publisher, the editors and the reviewers. Any product that may be evaluated in this article, or claim that may be made by its manufacturer, is not guaranteed or endorsed by the publisher.
Supplementary material
The Supplementary Material for this article can be found online at: https://www.frontiersin.org/articles/10.3389/fpls.2023.1104095/full#supplementary-material
References
Abbaspour, H., Pour, F. S. N., Abdel-Wahhab, M. A. (2021). Arbuscular mycorrhizal symbiosis regulates the physiological responses, ion distribution and relevant gene expression to trigger salt stress tolerance in pistachio. Physiol. Mol. Biol. Plants 27 (8), 1765–1778. doi: 10.1007/s12298-021-01043-w
Arab, Y., Marjan, Shahsavani, S., Akhyani, A., Dorostkar, V. (2018). Pomegranate growth affected by arbuscular mycorrhizae, phosphorus fertilizer, and irrigation water aalinity. Commun. Soil Sci. Plant Anal. 49 (4), 478–488. doi: 10.1080/00103624.2018.1431265
Assaha, D. V. M., Ueda, A., Saneoka, H., Al-Yahyai, R., Yaish, M. W. (2017). The role of na+ and k+ transporters in salt stress adaptation in glycophytes. Front. Physiol. 8 (1). doi: 10.3389/fphys.2017.00509
Athar, H., Zafar, Z. U., Ashraf, M. (2015). Glycinebetaine improved photosynthesis in canola under salt stress: evaluation of chlorophyll fluorescence parameters as potential indicators. J. Agron. Crop Sci. 201 (6), 428–442. doi: 10.1111/jac.12120
Chandrasekaran, M., Chanratana, M., Kim, K., Seshadri, S., Sa, T. (2019). Impact of arbuscular mycorrhizal fungi on photosynthesis, water status, and gas exchange of plants under salt stress-a meta-analysis. Front. Plant Sci. 10 (1). doi: 10.3389/fpls.2019.00457
Chen, Z., Chang, Y., Deng, C., Yang, Z. (2017). Effect of saline-alkali habitat on antioxidant enzyme activity biomass of sweet sorghum seedlings. J. Jilin Agric. Univ. 39 (01), 15–19. doi: 10.13327/j.jjlau.2016.2841
Diao, F., Dang, Z., Cui, X., Xu, J., Jia, B., Ding, S., et al. (2021). Transcriptomic analysis revealed distinctive modulations of arbuscular mycorrhizal fungi inoculation in halophyte Suaeda salsa under moderate salt conditions. Environ. Exp. Bot. 183 (1), 104337. doi: 10.1016/j.envexpbot.2020.104337
Dong, F. X., Wang, Y. H., Tang, M. (2021). Effects of Laccaria bicolor on gene expression of Populus trichocarpa root under poplar canker stress. J. Fungi (Basel) 7 (12), 1024. doi: 10.3390/jof7121024
Evelin, H., Devi, T. S., Gupta, S., Kapoor, R. (2019). Mitigation of salinity stress in plants by arbuscular mycorrhizal symbiosis: Current understanding and new challenges. Front. Plant Sci. 10 (1). doi: 10.3389/fpls.2019.00470
Fang, S., Hou, X., Liang, X. (2021). Response mechanisms of plants under saline-alkali stress. Front. Plant Sci. 12 (1). doi: 10.3389/fpls.2021.667458
Fazeli-Nasab, B., Piri, R., Rahmani, A. F. (2022). “Chapter 10 assessment of the role of rhizosphere in soil and its relationship with microorganisms and element absorption,” in Plant protection. Eds. Soni, R., Suyal, D. C., Goel, R. (Berlin, Boston: De Gruyter), 225–252. doi: 10.1515/9783110771558-010
Giovannetti, M., Mosse, B. (1980). An evaluation of techniques for measuring vesicular arbuscular mycorrhizal infection in roots. New Phytol. 84 (3), 489–500. doi: 10.1111/j.1469-8137.1980.tb04556.x
Huang, Z., Zou, Z., He, C., He, Z., Zhang, Z., Li, J. (2010). Physiological and photosynthetic responses of melon (Cucumis melo l.) seedlings to three Glomus species under water deficit. Plant Soil 339 (1), 391–399. doi: 10.1007/s11104-010-0591-z
Hui, R., Tan, H., Li, X., Wang, B. (2022). Variation of soil physical-chemical characteristics in salt-affected soil in the qarhan salt lake, qaidam basin. J. Arid Land 14 (3), 341–355. doi: 10.1007/s40333-022-0091-z
Jia, X., Zhu, H., Wang, H., Wu, Y., Zhao, T., Cheng, L., et al. (2019). Study on physiological response of Malus halliana to saline-alkali stress. Acta Ecol. Sin. 39 (17), 6349–6361. doi: 10.5846/stxb201804230919
Johnson, C. M., Stout, P. R., Broyer, T. C., Carlton, A. B. (1957). Comparative chlorine requirements of different plant species. Plant Soil 8 (4), 337–353. doi: 10.1007/BF01666323
Khan, Y., Shah, S., Hui, T. (2022). The roles of arbuscular mycorrhizal fungi in influencing plant nutrients, photosynthesis, and metabolites of cereal crops–a review. Agronomy 12 (9), 2191. doi: 10.3390/agronomy12092191
Kong, L., Gong, X., Zhang, X., Zhang, W., Sun, J., Chen, B. (2019). Effects of arbuscular mycorrhizal fungi on photosynthesis, ion balance of tomato plants under saline-alkali soil condition. J. Plant Nutr. 43 (5), 682–698. doi: 10.1080/01904167.2019.1701029
Kovar, M., Brestic, M., Sytar, O., Barek, V., Hauptvogel, P., Zivcak, M. (2019). Evaluation of hyperspectral reflectance parameters to assess the leaf water content in soybean. Water 11 (3), 443. doi: 10.3390/w11030443
Lim, S. J., Shin, M. N., Son, J. K., Song, J. D., Cho, K. H., Lee, S. H., et al. (2017). Evaluation of soil pore-water salinity using a decagon GS3 sensor in saline-alkali reclaimed tidal lands. Comput. Electron. Agric. 132 (2017), 49–55. doi: 10.1016/j.compag.2016.11.017
Liu, B., Hu, Y., Wang, Y., Xue, H., Li, Z., Li, M. (2022). Effects of saline-alkali stress on bacterial and fungal community diversity in Leymus chinensis rhizosphere soil. Environ. Sci. pollut. Res. Int. 29 (46), 70000–70013. doi: 10.1007/s11356-022-20270-6
Livak, K. J., Schmittgen, T. D. (2001). Analysis of relative gene expression data using real-time quantitative PCR and the 2–ΔΔCT method. Methods 25 (4), 402–408. doi: 10.1006/meth.2001.1262
Lu, K., GUo, S., Wei, Y., Zhang, C., Wu, N. (2018). Effects of exogenous 5-aminolevulinic acid on physiological characteristics and secondary metabolite contents of Atropa belladonna l. seedlings under NaCl stress. J. Southw. Univ. (Natural Sci. Edition) 40 (9), 13–20. doi: 10.13718/j.cnki.xdzk.2018.09.003
Luthfiana, N., Inamura, N., Tantriani, Sato, T., Saito, K., Oikawa, A., et al. (2021). Metabolite profiling of the hyphal exudates of Rhizophagus clarus and Rhizophagus irregularis under phosphorus deficiency. Mycorrhiza 31 (3), 403–412. doi: 10.1007/s00572-020-01016-z
Ma, Z., Zhao, X., He, A., Cao, Y., Han, Q., Lu, Y., et al. (2022b). Mycorrhizal symbiosis reprograms ion fluxes and fatty acid metabolism in wild jujube during salt stress. Plant Physiol. 189 (4), 2481–2499. doi: 10.1093/plphys/kiac239
Ma, S., Zhu, L., Wang, J., Liu, X., Jia, Z., Li, C., et al. (2022a). Arbuscular mycorrhizal fungi promote Gleditsia sinensis lam. root growth under salt stress by regulating nutrient uptake and physiology. Forests 13 (5), 688. doi: 10.3390/f13050688
Meng, K., Wu, Y. (2018). Footprints of divergent evolution in two Na+/H+ type antiporter gene families (NHX and SOS1) in the genus Populus. Tree Physiol. 38 (6), 813–824. doi: 10.1093/treephys/tpx173
Mohamed, I. A. A., Shalby, N., Bai, C., Qin, M., Agami, R. A., Jie, K., et al. (2020). Stomatal and photosynthetic traits are associated with investigating sodium chloride tolerance of Brassica napus l. cultivars. Plants (Basel) 9 (1), 62. doi: 10.3390/plants9010062
Neina, D. (2019). The role of soil pH in plant nutrition and soil remediation. Appl. Environ. Soil Sci. 2019 (1), 1–9. doi: 10.1155/2019/5794869
Phillips, J. M., Hayman, D. S. (1970). Improved procedures for clearing roots and staining parasitic and vesicular-arbuscular mycorrhizal fungi for rapid assessment of infection. Trans. Br. Mycol. Soc. 55 (1), 158–161. doi: 10.1016/S0007-1536(70)80110-3
Porcel, R., Aroca, R., Azcon, R., Ruiz-Lozano, J. M. (2016). Regulation of cation transporter genes by the arbuscular mycorrhizal symbiosis in rice plants subjected to salinity suggests improved salt tolerance due to reduced na+ root-to-shoot distribution. Mycorrhiza 26 (7), 673–684. doi: 10.1007/s00572-016-0704-5
Robin, A. H., Matthew, C., Uddin, M. J., Bayazid, K. N. (2016). Salinity-induced reduction in root surface area and changes in major root and shoot traits at the phytomer level in wheat. J. Exp. Bot. 67 (12), 3719–3729. doi: 10.1093/jxb/erw064
Santander, C., Aroca, R., Cartes, P., Vidal, G., Cornejo, P. (2021). Aquaporins and cation transporters are differentially regulated by two arbuscular mycorrhizal fungi strains in lettuce cultivars growing under salinity conditions. Plant Physiol. Biochem. 158 (2021), 396–409. doi: 10.1016/j.plaphy.2020.11.025
Smith, S. E., Facelli, E., Pope, S., F., A. S. (2009). Plant performance in stressful environments: interpreting new and established knowledge of the roles of arbuscular mycorrhizas. Plant Soil 326 (1), 3–20. doi: 10.1007/s11104-009-9981-5
Smith, S. E., Read, D. J. (2008). “Section I: Arbuscular mycorrhizas,” in Mycorrhizal symbiosis (Cambridge, Massachusetts: Academic press), 13–145. doi: 10.1016/B978-0-12-370526-6.X5001-6
Sui, N., Yang, Z., Liu, M., Wang, B. (2015). Identification and transcriptomic profiling of genes involved in increasing sugar content during salt stress in sweet sorghum leaves. BMC Genomics 16 (1), 534. doi: 10.1186/s12864-015-1760-5
Tian, F., Chang, E., Li, Y., Sun, P., Hu, J., Zhang, J. (2017). Expression and integrated network analyses revealed functional divergence of NHX-type Na+/H+ exchanger genes in poplar. Sci. Rep. 7 (1), 2607. doi: 10.1038/s41598-017-02894-8
Wang, Y. H., Dong, F. X., Tang, M. (2021). Transcriptome analysis of arbuscular mycorrhizal Casuarina glauca in damage mitigation of roots on NaCl stress. Microorganisms 10 (1), 15. doi: 10.3390/microorganisms10010015
Wang, X., Wang, Y., Song, Y., Dong, M. (2022b). Formation and functions of arbuscular mycorrhizae in coastal wetland ecosystems: A review. Ecosyst. Health Sustain. 8 (1), 2144465. doi: 10.1080/20964129.2022.2144465
Wang, F., Zhang, L., Zhou, J., Rengel, Z., George, T. S., Feng, G. (2022a). Exploring the secrets of hyphosphere of arbuscular mycorrhizal fungi: processes and ecological functions. Plant Soil 481 (2022), 1–22. doi: 10.1007/s11104-022-05621-z
Wu, N., Li, Z., Wu, F., Tang, M. (2016). Comparative photochemistry activity and antioxidant responses in male and female Populus cathayana cuttings inoculated with arbuscular mycorrhizal fungi under salt. Sci. Rep. 6 (1), 37663. doi: 10.1038/srep37663
Xun, F., Xie, B., Liu, S., Guo, C. (2015). Effect of plant growth-promoting bacteria (PGPR) and arbuscular mycorrhizal fungi (AMF) inoculation on oats in saline-alkali soil contaminated by petroleum to enhance phytoremediation. Environ. Sci. pollut. Res. Int. 22 (1), 598–608. doi: 10.1007/s11356-014-3396-4
Yang, Y., Geng, J., Yu, Q., Wang, J., Yu, W., Zhao, W. (2019b). Research progress on soil utilization and improvement in saline-alkali land. Agric. Technol. 39 (24), 108–111. doi: 10.19754/j.nyyjs.20191230039
Yang, X., Li, H., Jiang, L., Tang, X., Liu, X., Zhang, H. (2019a). Effects of arbuscular mycorrhiza fungi on the growth characteristics, root morphology, and ion distribution of Pyrus betulaefolia bunge under saline-alkaline stress. For. Sci. 66 (1), 97–104. doi: 10.1093/forsci/fxz047
Yang, Z., Li, J. L., Liu, L. N., Xie, Q., Sui, N. (2019c). Photosynthetic regulation under salt stress and salt-tolerance mechanism of sweet sorghum. Front. Plant Sci. 10 (1). doi: 10.3389/fpls.2019.01722
Yarra, R. (2019). The wheat NHX gene family: potential role in improving salinity stress tolerance of plants. Plant Gene 18 (2019), 100178. doi: 10.1016/j.plgene.2019.100178
Yarra, R., Kirti, P. B. (2019). Expressing class I wheat NHX (TaNHX2) gene in eggplant (Solanum melongena l.) improves plant performance under saline condition. Funct. Integr. Genomics 19 (4), 541–554. doi: 10.1007/s10142-019-00656-5
Yuan, Z., Pan, X., Jin, W. (2019). Tree-associated symbiotic microbes and underlying mechanisms of ecological interactions: a case study of poplar. Acta Ecol. Sin. 39 (01), 381–397. doi: 10.5846/stxb201710251909
Yu, M., Wu, Q., Zheng, D., Feng, N., Liang, X., Liu, M., et al. (2021). Plant growth regulators enhance saline–alkali tolerance by upregulating the levels of antioxidants and osmolytes in soybean seedlings. J. Plant Growth Regul. 41 (8), 3218–3232. doi: 10.1007/s00344-021-10507-y
Zhang, Y. (2020). Response to the growth physiology and PAL in Populus simonii × P. nigra under nitrogen treatment. [dissertation/master’s thesis]. [Harbin (Heilongjiang): Northeast Forestry University]. doi: 10.27009/d.cnki.gdblu.2020.000423
Zhang, Y., Kaiser, E., Marcelis, L. F. M., Yang, Q., Li, T. (2020b). Salt stress and fluctuating light have separate effects on photosynthetic acclimation, but interactively affect biomass. Plant Cell Environ. 43 (9), 2192–2206. doi: 10.1111/pce.13810
Zhang, M., Wang, M., Hou, S., Liu, J., Lin, P., Li, Y. (2020a). Effects of arbuscular mycorrhizal fungi on plant growth and secondary metabolism in Citrus reticulata. J. Trop. Subtrop. Bot. 28 (1), 78–83. doi: 10.11926/jtsb.4087
Zhou, Z. (2022). Metabolomics analysis of Medicago Sativa L. in response to saline and alkali stress. [dissertation/master’s thesis]. [Harbin (Heilongjiang): Harbin Normal University]. doi: 10.27064/d.cnki.ghasu.2022.001469
Zhou, Y., Wang, S., Liu, Y., Li, K., You, X. (2019). Sequencing and assembly of Populus simonii × P. nigra genome and SSR analysis. Bull. Bot. Res. 39 (1), 156–160. doi: 10.7525/j.issn.1673-5102.2019.01.019
Zhou, Y., Yin, X., Wan, S., Hu, Y., Xie, Q., Li, R., et al. (2018). The Sesuvium portulacastrum plasma membrane Na+/H+ antiporter SpSOS1 complemented the salt sensitivity of transgenic Arabidopsis sos1 mutant plants. Plant Mol. Biol. Rep. 36 (4), 553–563. doi: 10.1007/s11105-018-1099-6
Zhu, Y., Xiong, J., Lv, G., Batool, A., Wang, Z., Li, P., et al. (2015). Effects of arbuscular mycorrhizal fungi and plant symbiosis on plant water relation and its mechanism. Acta Ecol. Sin. 35 (8), 2419–2427. doi: 10.5846/stxb201306091556
Zorb, C., Noll, A., Karl, S., Leib, K., Yan, F., Schubert, S. (2005). Molecular characterization of Na+/H+ antiporters (ZmNHX) of maize (Zea mays l.) and their expression under salt stress. J. Plant Physiol. 162 (1), 55–66. doi: 10.1016/j.jplph.2004.03.010
Keywords: saline-alkali stress, arbuscular mycorrhizal fungi, Populus simonii×P. nigra, NHX gene family, Funneliformis mosseae
Citation: Dong F, Wang Y, Tao J, Xu T and Tang M (2023) Arbuscular mycorrhizal fungi affect the expression of PxNHX gene family, improve photosynthesis and promote Populus simonii×P. nigra growth under saline-alkali stress. Front. Plant Sci. 14:1104095. doi: 10.3389/fpls.2023.1104095
Received: 22 November 2022; Accepted: 16 January 2023;
Published: 30 January 2023.
Edited by:
Ece Turhan, Eskişehir Osmangazi University, TürkiyeReviewed by:
Dejian Zhang, Yangtze University, ChinaMohd Asgher, Baba Ghulam Shah Badshah University, India
Copyright © 2023 Dong, Wang, Tao, Xu and Tang. This is an open-access article distributed under the terms of the Creative Commons Attribution License (CC BY). The use, distribution or reproduction in other forums is permitted, provided the original author(s) and the copyright owner(s) are credited and that the original publication in this journal is cited, in accordance with accepted academic practice. No use, distribution or reproduction is permitted which does not comply with these terms.
*Correspondence: Ming Tang, tangmingyl@163.com; Tingying Xu, tingying.xu@okstate.edu