Corrigendum: Cardioprotective effect of Sanguisorba minor against isoprenaline-induced myocardial infarction in rats
- 1Department of Pharmacology, Faculty of Medicine, Mashhad University of Medical Sciences, Mashhad, Iran
- 2Pharmacological Research Center of Medicinal Plants, Mashhad University of Medical Sciences, Mashhad, Iran
- 3Department of Physiology, Faculty of Medicine, Mashhad University of Medical Sciences, Mashhad, Iran
- 4Department of Internal Medicine, Faculty of Medicine, Mashhad University of Medical Science, Mashhad, Iran
- 5Department of Pathology, Faculty of Medicine, Mashhad University of Medical Sciences, Mashhad, Iran
- 6Biotechnology Research Center, Pharmaceutical Technology Institute, Mashhad University of Medical Sciences, Mashhad, Iran
- 7Applied Biomedical Research Center, Mashhad University of Medical Sciences, Mashhad, Iran
- 8Department of Basic Medical Sciences, College of Medicine, QU Health, Qatar University, Doha, Qatar
Introduction: Oxidative stress is a major instigator of various cardiovascular diseases, including myocardial infarction (MI). Despite available drugs, there is still an increased need to look for alternative therapies or identify new bioactive compounds. Sanguisorba minor (S. minor) is a native herb characterized by its potent antioxidant activity. This study was designed to evaluate the effect of S. minor against isoprenaline-induced MI.
Methods: Rats were treated with the hydro-ethanolic extract of the aerial parts of S. minor at doses of 100 or 300 mg/kg orally for 9 days. Isoprenaline was injected subcutaneously at the dose of 85 mg/kg on days 8 and 9. Then, the activities of various cardiac injury markers including cardiac troponin (cTnT), lactate dehydrogenase (LDH), creatinine kinase muscle brain (CK-MB), creatinine phosphokinase (CPK), and antioxidant enzymes in serum were determined. Malondialdehyde (MDA) and thiol content were measured in cardiac tissue, and histopathological analysis was conducted.
Results: Our results show that isoprenaline increased the serum levels of cTnT, LDH, CK-MB, and CPK (p < 0.001) and elevated MDA levels (p < 0.001) in cardiac tissue. Isoprenaline also reduced superoxide dismutase (SOD), catalase, and thiol content (p < 0.001). Importantly, the extract abolished isoprenaline-induced MI by elevating SOD and catalase (p < 0.001), reducing levels of MDA, and diminishing levels of cTnT, LDH, CK-MB, and CPK cardiac markers (p < 0.001). Histopathological studies of the cardiac tissue showed isoprenaline-induced injury that was significantly attenuated by the extract.
Conclusion: Our results suggest that S. minor could abrogate isoprenaline-induced cardiac toxicity due to its ability to mitigate oxidative stress.
1 Introduction
Cardiovascular disease (CVD) continues to be a leading cause of morbidity and mortality despite advances in modern medicine. Ischemic heart disease and myocardial infarction (MI) are more common than other CVDs. MI, in particular, is a major contributor to CVD-associated mortality. That is not surprising as MI causes irreversible structural and functional damage to the cardiac tissue (Surekha et al., 2007; Ansari et al., 2019).
There is an increased appreciation of medicinal plants and their phytochemicals both in pharmacological studies and drug development programs (Al Dhaheri et al., 2014; El Hasasna et al., 2015; El Hasasna et al., 2016; Athamneh et al., 2017; Alsamri et al., 2019; Badran et al., 2019; Alsamri et al., 2021a; Mesmar et al., 2021; AlKahlout et al., 2022; Alsamri et al., 2022). Approximately, a third of all drugs approved by the Food and Drug Administration (FDA) over the past 20 years have been obtained from or inspired by natural/herbal compounds/derivatives (Izzo et al., 2020). In traditional medicine, there are several natural products such as extracts, herbomineral, polyherbal formulations, and crude herbals, which can be used for CVD (Upaganlawar et al., 2011; Anwar et al., 2018; Hosseini et al., 2022). For example, the cardioprotective activities of some phytochemicals such as silymarin, quercetin, curcumin, berberine, naringenin, baicalin, resveratrol, and myricitrin have been well documented in various studies (Rao and Viswanath, 2007; Giannouli et al., 2018; Hesari et al., 2021). These beneficial effects are due to the presence of a broad spectrum of antioxidative, antiangiogenic, anti-ischemic, anti-thrombotic, and anti-inflammatory activities (Hesari et al., 2021). Among the attractive reasons for utilizing these natural products are their documented fewer side effects, ease of access, and affordability (Upaganlawar et al., 2011; Al Disi et al., 2015; Anwar et al., 2016; Samaha et al., 2019; Salama et al., 2020).
There are various approaches that could be employed to mimic MI in the laboratory. Examples include ligating the coronary artery (Thang et al., 2018) or administration of a high dose of isoprenaline (ISO), a β-adrenergic agonist. The advantage of using ISO is that it is a non-invasive method that has been shown to exert damage to cardiac myocytes via various mechanisms including hypoxia, calcium overload, production of free radicals, and energy depletion (Patel et al., 2010).
Different studies have shown that medicinal herbs or their active compounds can attenuate ISO-induced MI by virtue of their antioxidative capacities (Upaganlawar and Balaraman, 2011). One plant that appears to possess several bioactives which are potent antioxidants is Sanguisorba. minor (S. minor). This plant belongs to the Rosaceae family and is used in traditional medicine for bleeding, eczema, diarrhea, etc. (Zhao et al., 2017). Reports show that several phytochemicals such as phenols, flavonoids, and terpenoids can be isolated from the Sanguisorba genus and are known to abate oxidative milieu (Zhao et al., 2017). In particular, the antioxidant activity of S. minor is owed primarily to its bountiful polyphenols. Moreover, the pharmacological profile of S. minor includes anti-bacterial, neuroprotective, anti-inflammatory, and anti-cancer properties (Zhao et al., 2017; Finimundy et al., 2020; Ćirović et al., 2021). Given the antioxidant activity of various bioactives in S. minor, we undertook this study to determine the potential effects of S. minor against ISO-induced MI in rats.
2 Materials and methods
2.1 Reagents
Isoprenaline, trichloroacetic acid (TCA), 2-thiobarbituric acid (TBA), ketamine, xylazine hydrochloride, and 3-(4,5-dimethylthiazol-2-yl) 2,5-diphenyltetrazolium bromide (MTT) were bought from Sigma-Aldrich (St. Louis, MO, United States). 5,5′-Dithiobis-(2-nitrobenzoic) acid (DTNB) and pyrogallol were obtained from Cayman (Michigan, United States).
2.2 Preparation of extracts
S. minor was obtained from Quchan, Iran (37.1008° N, 58.5054° E). The collected plant was identified by M.R. Joharchi from the Ferdowsi University of Mashhad. The voucher specimen (No. 45489) was recorded and deposited in the Herbarium of the School of Agriculture, Ferdowsi University of Mashhad, Mashhad, Iran. To this end, we used the aerial parts of the S. minor which were collected in the spring.
After drying the plant, the aerial parts were ground to fine powder, and 50 g of the powder was soaked in 200 mL of a hydro-ethanolic solution (50%, v/v) for 48 h at 40°C. Afterward, the extract was dried using a rotary vacuum evaporator at 37 °C (Norouzi et al., 2019). The yield of extract was 20% (w/w).
2.3 Animals
Adult male Wistar rats weighing 200–250 g were obtained from the Animal Research Center of the Faculty of Medicine, Mashhad University of Medical Sciences, Mashhad, Iran. Rats were kept in a temperature-controlled environment (22 ± 4°C) with a 12 h day/night cycle. Animals had free access to food and tap water ad libitum. All experiments were performed in accordance with the National Institutes of Health (NIH) Guide for the Care and Use of Laboratory Animals and were approved by the Animal Ethics Committee of Mashhad University of Medical Sciences, Mashhad, Iran (IR.MUMS.MEDICAL.REC.1399.426).
2.4 Stimulation of MI
MI was induced by administration of ISO (subcutaneously; SC) at the dose of 85 mg/kg daily for 2 days (on days 8 and 9) as previously published (El-Gohary and Allam, 2017).
2.5 Experimental protocol
Rats were divided into five groups, each comprising six animals. Saline was gavaged to the control group for 9 days. The ISO group received normal saline for 9 days; ISO was injected SC (0.3 mL) on days 8 and 9. The experimental/therapeutic groups received hydroalcoholic extract orally at the doses of 100 and 300 mg/kg for 9 days; ISO was injected SC (0.3 mL) on days 8 and 9 as performed with the control group. To assess the potential toxicity of the extract, one group of animals received a high dose of the extract (300 mg/kg) for 9 days. For collecting blood samples from the heart, rats were anesthetized with ketamine/xylazine on the last day, and then, the heart tissue was immediately separated and frozen in liquid nitrogen. Tissue and serum samples were stored at −80 °C for further biochemical analyses and histopathological studies (Figure 1).
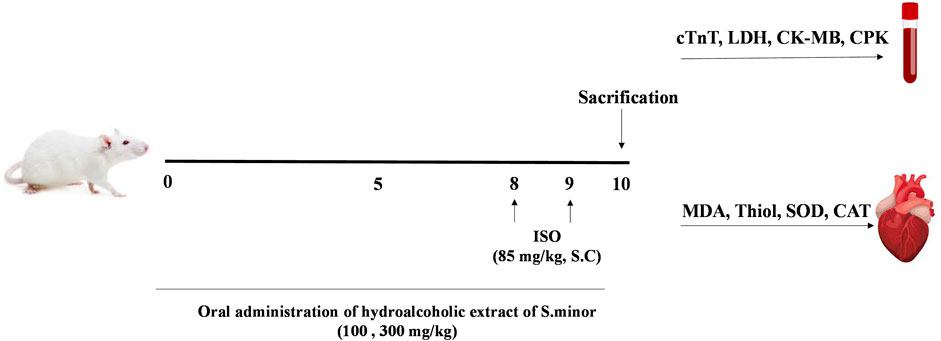
FIGURE 1. Diagrammatic sketch showing the experimental protocol. ISO: isoprenaline, cTnT: cardiac troponin, LDH: lactate dehydrogenase, CK-MB: creatinine kinase muscle brain, CPK: creatinine phosphokinase, MDA: malondialdehyde, and SOD: superoxide dismutase.
2.5.1 Measurement of malondialdehyde
Lipid peroxidation was evaluated by the determination of malondialdehyde (MDA) in cardiac tissue as previously published (Boroushaki et al., 2019). In brief, trichloroacetic acid (10%) and thiobarbituric acid (0.67%) were added to the sample and boiled for 40 min. HCl and n-butanol were added to cooled samples. After centrifugation, the absorbance of the supernatant was read at 535 nm. Furthermore, the concentration of MDA was determined as equal to absorbance/(1.56*105 cm−1M−1).
2.5.2 Determination of thiol contents
The thiol content was measured according to the work of Hosseini et al. (2017). Then, 50 μL of tissue homogenate was mixed with Tris-EDTA buffer (pH = 8.6), and absorbance was measured at 412 nm (A1). 5,5′-Dithiobis-(2-nitrobenzoic) acid (DTNB) was added to the solution, and then, the absorbance after 15 min was determined (A2). DTNB was used as blank (B). The equation used to quantitate the amount of thiol content is total thiol concentration (mM) = ((A2-A1-B)*0.7)/0.05*14.
2.5.3 Determination of catalase activity
Catalase (CAT) activity was measured according to the Aebi protocol (Aebi, 1984). This method is based on the constant rate (k) of hydrogen peroxide destruction detection by measuring its reduction in absorbance at 240 nm/min. The catalase activity was measured as K (constant)/L.
2.5.4 Evaluation of superoxide dismutase activity
The activity of superoxide dismutase (SOD) was determined as previously published (Madesh and Balasubramanian, 1998). In brief, this is a colorimetric assay involving superoxide production by pyrogallol auto-oxidation. Suppression of superoxide-dependent reduction of the MTT by SOD was calculated at 570 nm. A unit of SOD activity is defined as the amount of enzyme that induces 50% inhibition in the MTT reduction rate (Madesh and Balasubramanian, 1998).
2.5.5 Measurement of enzyme markers
The amount of lactate dehydrogenase (LDH), creatinine kinase (CPK), cardiac troponin (cTn-T), creatinine kinase-MB (CK-MB), ALT, and AST was measured in serum according to standard kits as previously published (Lewandrowski et al., 2002).
2.5.6 Measurement of lipid profile
The number of lipids such as total cholesterol (TC), triglyceride (TG), very low-density lipoprotein (VLDL), low-density lipoprotein (LDL), and high-density lipoprotein (HDL) was measured in serum as per the manufacturer’s protocol (Pars Azmun Co., Karaj, Iran).
2.5.7 Histopathological investigation
Formalin (10%) was used to fix isolated cardiac for histopathological studies. The tissues were paraffinized and cut into 3–4 mm slices. Hematoxylin and eosin (H&E) staining was performed, and sections were visualized by light microscopy.
2.6 Statistical analysis
All data are expressed as mean ± SEM. One-way ANOVA followed by the Tukey–Kramer test was performed, and a p < 0.05 was considered significant.
3 Results
3.1 Effect of S. minor on lipid peroxidation and thiol content
MDA is considered a lipid peroxidation index. As expected, treatment with ISO increased the level of MDA following MI (###p < 0.001). S. minor extract significantly reduced the level of MDA at the doses of 100 and 300 mg/kg (**p < 0.01, ***p < 0.001) (Figure 2). On the other hand, ISO decreased thiol content (###p < 0.001) in cardiac tissue, while S. minor (300 mg/kg) increased the total thiol content (***p < 0.001) (Figure 1). Importantly, the level of MDA and thiol in the extract-only group (i.e., the group without ISO) was similar to that in the control group (Figure 2). Taken together, these findings argue for a cardioprotective effect of S. minor against ISO-induced lipid peroxidation or thiol suppression.
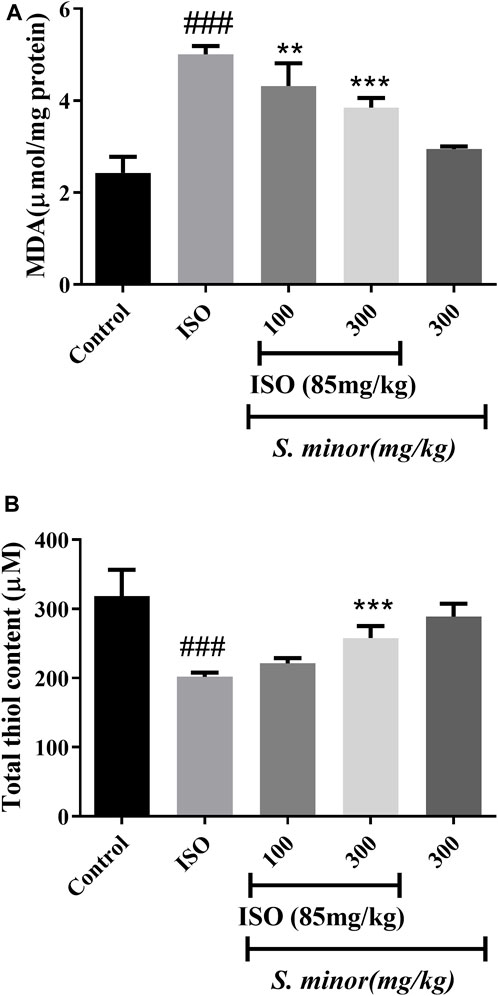
FIGURE 2. Effect of S. minor on the malondialdehyde level and thiol content in heart tissue after isoprenaline administration. The level of MDA (A) and thiol (B) content was evaluated in heart tissue. Data were expressed as mean ± SEM. ###p < 0.001 in comparison with the control group. **p < 0.01 and ***p < 0.001 in comparison with isoprenaline (85 mg/kg).
3.2 Effect of S. minor on antioxidant enzymes
As shown in Figure 3, the level of the antioxidant enzymes, SOD and CAT, was attenuated following ISO administration (###p < 0.001). Importantly, oral pretreatment with S. minor at the doses of 100 and 300 mg/kg (**p < 0.01, ***p < 0.001). This result shows that the S. minor extract abrogates ISO-induced suppression of antioxidant defense mechanisms.
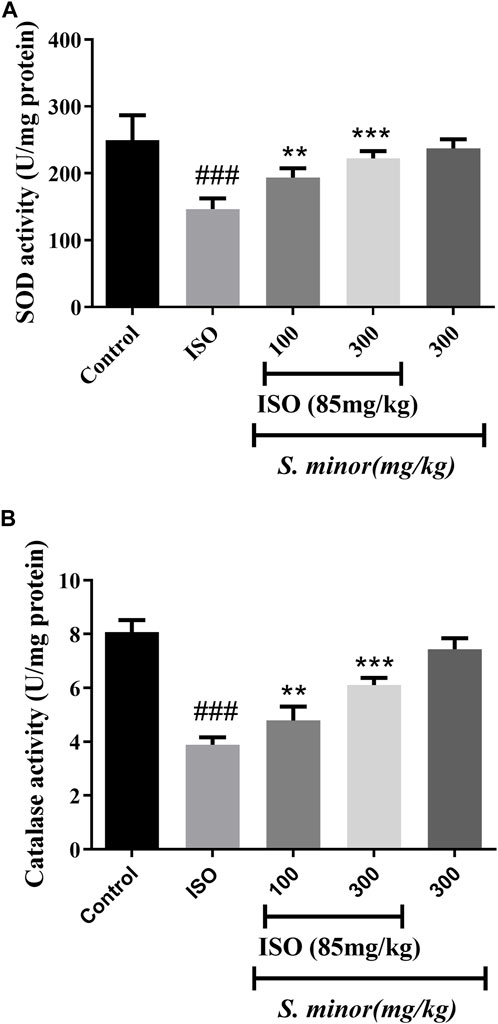
FIGURE 3. Effect of S. minor on the activity of superoxide dismutase and catalase after isoproterenol administration. The activity of SOD (A) and CAT (B) was evaluated in tissue. Data were expressed as mean ± SEM. ###p < 0.001 in comparison with the control group. **p < 0.01 and ***p < 0.001 in comparison with isoprenaline (85 mg/kg).
3.3 Effect of S. minor on cardiac injury markers
Compared with the control group, animals treated with ISO exhibited significant elevation in serum levels of cTn-T, CPK, CKMB, and LDH (Figure 4) (###p < 0.001). Importantly, both doses (100 and 300 mg/kg) of the extract significantly reduced the level of CPK (***p < 0.001) and LDH (***p < 0.001). The levels of CKMB and cTn-T were decreased significantly by the extract at the dose of 300 mg/kg (***p < 0.001). Importantly, the extract alone did not change the level of cardiac injury markers. Thus, these results argue for an ameliorative effect of the S. minor extract against cardiac injury.
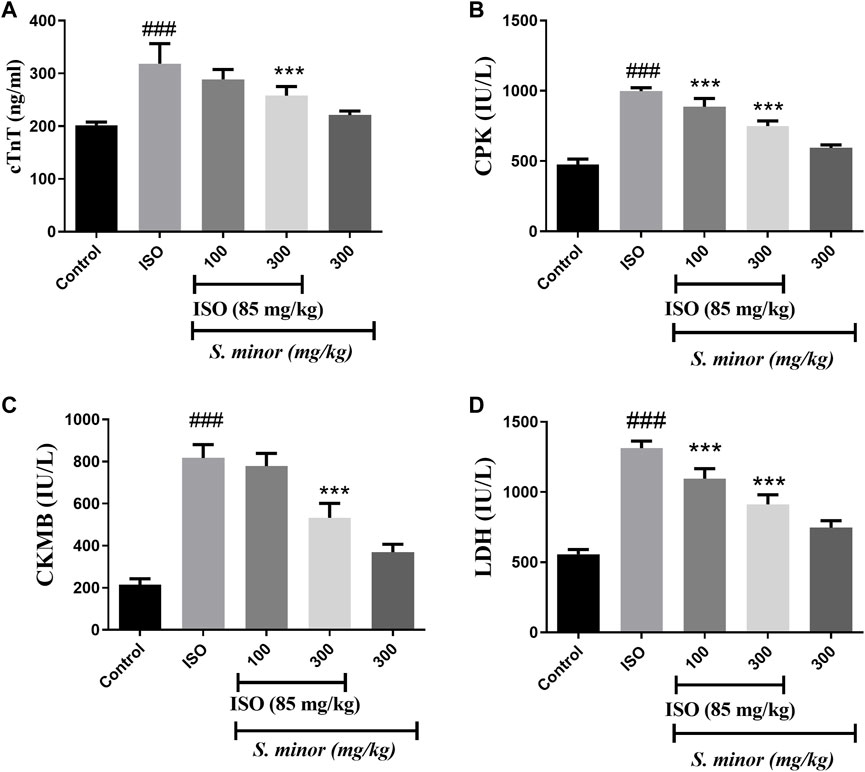
FIGURE 4. Effect of S. minor on the levels of cTn-T, CK-MB, LDH, and CPK after isoproterenol administration. The levels of cTn-T (A), CK-MB (C), CPK (B), and LDH (D) were evaluated in serum. Data were expressed as mean ± SEM. ###p < 0.001 in comparison with the control group. ***p < 0.001 in comparison with isoprenaline (85 mg/kg).
3.4 Effect of S. minor on liver enzymes
The serum levels of AST and ALT were measured in serum as shown in Figure 5. ISO caused a dramatic increase in both enzymes in comparison with the control group (###p < 0.001). Importantly, 100 mg/kg and 300 mg/kg (***p < 0.001) of S. minor attenuated the ISO-induced increase of AST and ALT (Figure 5). It is worth mentioning that the high dose of S. minor did not elicit any effects on the levels of both enzymes in the animals treated with the extract alone (i.e., absent of ISO) (Figure 5).
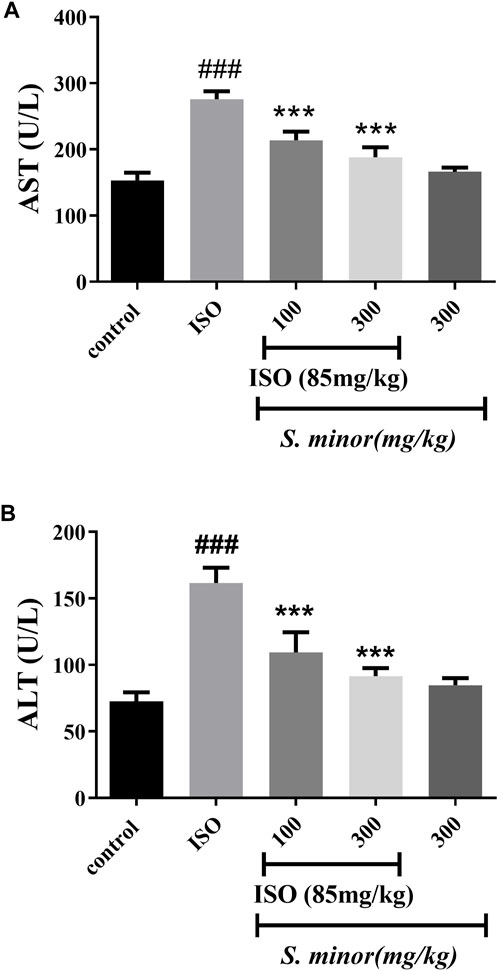
FIGURE 5. Effect of S. minor on the levels of AST (A) and ALT (B) after isoproterenol administration. The levels of these enzymes were evaluated in serum. Data were expressed as mean ± SEM. ###p < 0.001 in comparison with the control group. ***p < 0.001 in comparison with isoprenaline (85 mg/kg).
3.5 Effect of S. minor on the lipid profile
Our results (Table 1) show that ISO increased (###p < 0.001) the level of serum lipids following MI induction and decreased the amount of HDL in serum. Oral pretreatment with 300 mg/kg (***p < 0.001) of the extract reduced the amount of TG, TC, LDL, and VLDL, and increased HDL in comparison with the ISO group. A similar effect was noted for 100 mg/kg (*p < 0.05) except that TC was not affected by this dose. Importantly, treatment of S. minor alone (i.e., without ISO) did not modify the lipid profile (Table 1). Thus, S. minor exerts a favorable effect on the dysregulated lipid profile induced by ISO.
3.6 Histopathological effects of S. minor
The microscopic evaluation of the ISO-treated group revealed marked cellular damage including cytoplasmic hypereosinophilia, nuclear shrinkage, edema, congestion, small foci of hemorrhage, and foci of inflammatory cell infiltration composed of macrophages, lymphocytes, and neutrophils (Figure 6). Oral pretreatment with the extract at the dose of 300 mg/kg showed less-pronounced changes including mild edema and congestion, but not hemorrhage, and scarce foci of mild inflammatory reaction (Figure 6). Importantly, treatment with the extract alone at a dose of 100 mg/kg showed some edema and inflammatory infiltrates, while the higher dose (300 mg/kg) exhibited only very mild inflammatory infiltration.
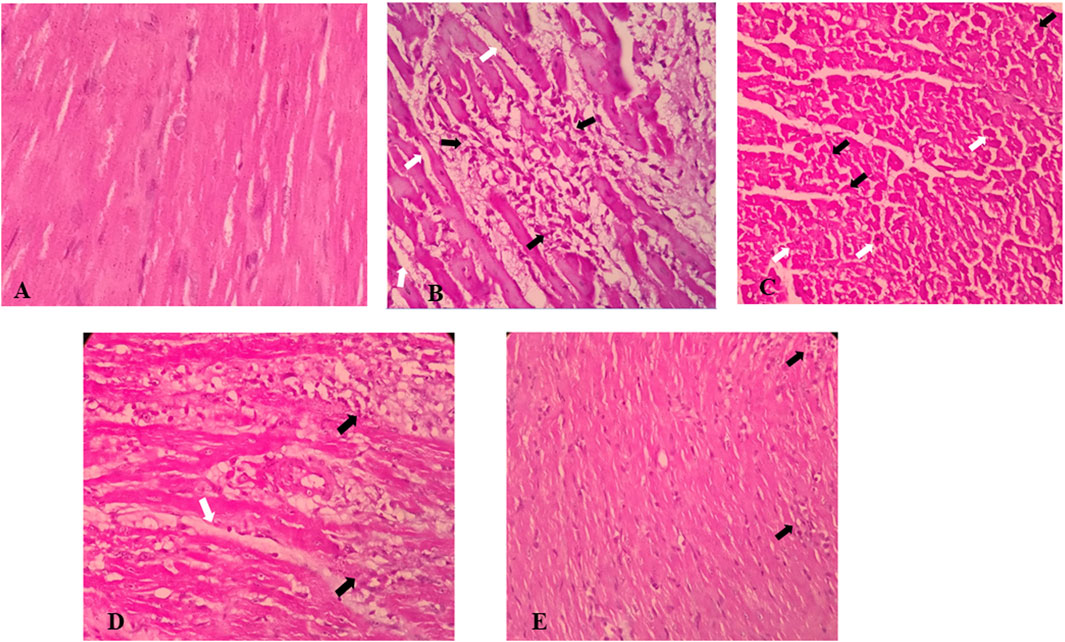
FIGURE 6. Effects of S. minor on histological changes of heart tissue in rats. (A) Control group (×400, H&E), (B,C) isoprenaline group shows edema (white arrows) and intense inflammatory infiltration (black arrows) (B) (×400, H&E) and cellular damage including cytoplasmic hyper eosinophilia (white arrows) and nuclear shrinkage (black arrows) (C) (×400, H&E), and (D) isoprenaline + 100 mg/kg extract shows edema (white Arrow) and severe inflammatory infiltrate (black Arrow), similar to the isoprenaline group (×400, H&E), while isoprenaline + 300 mg/kg extract [(E) ×400, H&E] shows very milder inflammatory infiltration (black arrows).
4 Discussion
In this report, we employed ISO as an agent to induce a phenotype similar to MI. Our findings showed that ISO increased the levels of TC, TG, LDL-C, and VLDL-C and reduced the amount of HDL-C. This result is in agreement with previous reports showing a dyslipidemic effect of an ISO insult (Manjula et al., 1992). The elevation of lipids in serum following ISO administration in rats might be due to increased mobilization of lipids from adipose tissue or via increased cyclic adenosine monophosphate (Strålfors et al., 1984; Paritha and Shyamala, 1997). Interestingly, recent studies have reported that plants from the Rosacea family can play an ameliorative role in the modulation of the lipid profile (Klevanova and Trzhetsynskiy, 2015; Jung et al., 2016).
In this study, we showed that pretreatment with the extract of S. minor, a member of the Rosacea family, significantly reduced serum levels of TC, TG, LDL-C, and VLDL-C, while, at the same time, increasing levels of HDL-C. This favorable modulate of lipidemia by S. minor supports its utilization for such an effect. To the best of our knowledge, this is the first study to report the hypolipidemic effects of S. minor. Interestingly, however, several studies have previously reported the hypolipidemic effects of quercetin and ellagic acid, two bioactives of S. minor. Similarly, studies of other Sanguisorba species such as Sanguisorba officinalis also alluded to a hypolipidemic effect. For instance, Mai et al., showed that ellagic acid reduced the lipid profile following ISO administration via HMG-CoA reductase inhibition (Elhemely et al., 2014). Likewise, quercetin decreased the lipid profile following doxorubicin (Aziz, 2021a), and the S. officinalis extract modulated the lipid profile in obese mice (Zheng et al., 2023). Based on these studies, it is reasonable to assume that the hypolipidemic effect of S. minor is due to its bountiful content of many bioactives, including quercetin and ellagic acid. Although these protective effects of S. minor are in accordance with the reported anti-hyperlipidemia effect of this plant family, further research is still needed to better determine and elucidate the underlying mechanisms of S. minor.
CK-MB and LDH are two diagnostic markers that are commonly used to determine cardiac injury. They are typically present in the cellular compartment and play a significant role in proper gradients for essential ions across cellular membranes within the myocytes (Preus et al., 1988). Moreover, it is well established that alterations in the properties of these enzymes impart a significant effect on the function of the heart. Contextually, during myocardial damage, CK-MB and LDH leak out into the circulation due to the disintegration of cardiomyocytes (Mari Kannan and Darlin Quine, 2011). It is the stabilization of the sarcolemma that protects cardiomyocytes against injury and, hence, does not allow for dramatic cell damage and release of the aforementioned enzymes. Moreover, as expected, ISO caused a dramatic increase in the levels of several enzymes, namely, CK-MB, CPK, LDH, AST, and ALT. Previous studies have revealed cardiomyocyte injury, resulting from glucose or oxygen deprivation, robustly modified membrane permeability and promoted leakage of these enzymes (Todd et al., 1980). In addition, the cardiac cytosolic calcium level is a key factor involved in maintaining normal activity levels of cardiac enzymes. ISO-induced myocardial necrosis has been reported to enhance adenylate cyclase activity, resulting in increased formation of cAMP and elevation of the cardiac cytosolic calcium level (Suchal et al., 2016).
The levels of LDH, CK-MB, and CK increase after MI and decrease to a normal range within 24 h. Total CK and CK-MB could be related to infarct size and can be used as predictors of prognosis. Elevation of these markers is intimately associated with cell membrane rupture; therefore, stabilization of the membrane by natural products or phytochemicals can have a role in the attenuation of these markers (Aydin et al., 2019). In this study, S. minor decreased these markers following ISO-induced MI. We previously reported the characterization of the S. minor extract by liquid chromatography–mass spectrometry, which confirmed the presence of quercetin, myricetin, kaempferol, catechin, ellagic acid, and gallic acid derivatives. Here, we show that S. minor can be effective in ameliorating the induced infarction, which could be due to the presence of different bioactive ingredients such as quercetin (Panda and Kar, 2015), kaempferol (Vishwakarma et al., 2018a), myricetin (Tiwari et al., 2009), catechin (Devika and Prince, 2008), and ellagic acid (Warpe et al., 2015). These and other flavonoids are well known to exert various beneficial effects including cardiovasculoprotective ones (Fardoun et al., 2019; Maaliki et al., 2019; Fardoun et al., 2020; Alsamri et al., 2021b; Slika et al., 2022). In particular, the cardioprotective effects of quercetin have been widely documented in both in vitro and in vivo experimental models of cardiac injury. Quercetin exerts cardioprotection in ischemia–reperfusion injury, autoimmune myocarditis, ISO- and doxorubicin-induced cardiotoxicities, and diabetic cardiomyopathy (Arumugam et al., 2012; Chen et al., 2013; Wang et al., 2013; Cote et al., 2015; Kumar et al., 2017). Numerous mechanisms such as suppression of oxidative stress, inhibition of inflammation and apoptosis, and affecting intracellular protein kinase cascades are involved in the cardioprotective potential of quercetin (Li et al., 2016; Ferenczyova et al., 2020). Kaempferol, a flavonoid that is bountiful in S. minor, could protect against cardiotoxicity precipitated by angiotensin II (Du et al., 2019), cisplatin (Qi et al., 2020), 5-fluorouracil (Safarpour et al., 2022), ISO (Vishwakarma et al., 2018b), or doxorubicin (Xiao et al., 2012). This protection occurs by virtue of the antiapoptotic, antioxidative, anti-inflammatory, calcium regulatory, and antifibrotic mechanisms of kaempferol (Kamisah et al., 2023). Likewise, ellagic acid, another important component of S. minor, showed cardioprotection against ISO-induced MI by the inhibition of oxidative stress and improvement of cardiac enzymes (Kannan and Quine, 2011).
CTn-T is a contractile protein that controls the calcium-mediated interaction of actin and myosin, which results in contraction and relaxation of striated muscle (Mari Kannan and Darlin Quine, 2011). This protein is not typically expressed in high levels under physiological conditions; however, its levels markedly increased following cardiac necrosis or infarction (Weil et al., 2017). The level of cTn-T after MI has been correlated with the severity of infarction in experimental animal models and contemplated for early detection of cardiotoxic potential in rodents. ISO-caused oxidative damage to the myocardium changes the cell membrane permeability and leads to cTn-T cardiac enzyme leakage to the circulation (Zhang et al., 2008). Here, ISO, and rather expectedly, increased the level of cTn-T, indicative of cellular injury, which was confirmed by Bertinchant et al. (2000). Pretreatment with the extract greatly diminished the increased cTn-T levels and, thus, helped prevent further excessive damage by preserving the structural integrity of the cells.
Oxidative stress plays a critical role in the onset and pathogenesis of many diseases, including CVD (Al Dhaheri et al., 2014; Shouk et al., 2014; Thuan et al., 2018; Alsamri et al., 2019; Mesmar et al., 2021; Shaito et al., 2022; Slika et al., 2022; Aramouni et al., 2023). Our study investigated the level of MDA as an oxidative stress index increased in rats that received ISO. The extract dramatically abrogated the ISO-induced MDA, strongly suggestive of the potent antioxidant activity of S. minor. This attenuation by the extract might be due to the elevation of the antioxidant activity of enzymes such as SOD and CAT (Cristina et al., 2021; Ćirović et al., 2021). Superoxide is an antioxidant enzyme that catalyzes the dismutation reaction of superoxide radicals (McCord and Fridovich, 1969). Contextually, the activity of SOD was reduced in ISO-treated rats, while pretreatment with extract restored the activity of SOD. This is in accordance with the report of Shiny et al. (2005) and Shen et al. (2019). Likewise, the activity of catalase decreased in ISO-treated rats in comparison with other groups. The activity of catalase increased following administration of the extract, indicating the antioxidant effects and free radical scavenging potential of the S. minor extract (Ćirović et al., 2021). It is well known that MI leads to the accumulation of superoxide radicals in the damaged site, imparting further necrotic effects on the myocardium (Saravanan and Prakash, 2004). Taken together, this shows that the extract decreases the depletion of antioxidant enzymes and, hence, exerts a protective effect against ISO-induced oxidative milieu.
The microscopic evaluation of the ISO-treated group revealed marked cellular damage, edema, congestion, small foci of hemorrhage, and foci of inflammatory cell infiltration composed of macrophages, lymphocytes, and neutrophils. Oral pretreatment of extract at high dose showed less-pronounced changes including mild edema and congestion, but not hemorrhage and scars foci of mild inflammatory reaction. In accordance with our findings, others have shown that ISO induces degenerative changes in heart tissue (Zaafan et al., 2013; Yaseen et al., 2017) and that quercetin improves histopathologic alterations induced by ISO (Zaafan et al., 2013) or doxorubicin (Aziz, 2021b).
The biochemical findings were confirmed by histopathological studies. ISO stimulated degenerative myocardium, inflammation, and hemorrhage which led to more release of cardiac markers, while pretreatment with S. minor indicated regenerative effects and attenuated pathological changes. As such, the favorable modulation of the biochemical parameters discussed herewith may, at least partly, explain these beneficial effects of S. minor observed in the histopathological findings. Although the present findings provide promising evidence as to the cardioprotective effects of S. minor against MI, there are limitations deserving acknowledgment. Assessment of cardiac function, e.g., through echocardiography, was not conducted in this study. In addition, assessment of cardiac stress, inflammation, fibrosis, and endothelial function indices could have added further insights as to the mechanisms underlying the cardioprotective effects of S. minor during ISO-induced MI. In a rat ISO model of cardiotoxicity, overstimulation of β-receptors evokes a decrease in the cardiac output and stroke volume and impairs systolic and diastolic functions in echocardiography (Filipský et al., 2012). As such, future studies addressing these limitations may provide better insights into the holistic benefits of S. minor in cardiovascular disease, particularly ISO-induced cardiac damage (Filipský et al., 2012).
Finally, it is particularly important here to mention that the content of bioactive compounds varies according to several factors including plant organ, phenological stage, growing regions, climatic patterns, and temperature. For instance, the aerial parts of S. minor (leaves and stems) have a high flavonoid content, with quercetin and ellagic acid being predominant, while they are less abundant in roots (Tocai Moţoc et al., 2023). The level of phenolic compounds during spring–summer seasons is higher than in other seasons, which may be related to the production of new current-year leaves (Karkanis et al., 2019). Therefore, careful attention to the content would become important for pharmacotherapeutic applications.
5 Conclusion
It is evident from the current study that oral pretreatment with S. minor modulated oxidative stress and reduced ISO-induced cardiac injury (Figure 7). The cardioprotective effects of S. minor may be linked to the bountiful presence of antioxidant bioactives, such as quercetin, myricetin, kaempferol, catechin, ellagic acid, and gallic acid, in this plant. This supports the traditional use of the plant and adds one more piece of evidence for its incorporation in complementary and alternative approaches in the war against CVD. Given the seasonal variability in the content of the extract and the aforementioned factors contributing to such contents, caution must be taken as the generalizability of findings might be affected by these factors. This necessitates further research on the identification and purification of active ingredients of the plant. Moreover, further studies are certainly needed to absorb this plant or its bioactives into clinical settings.
Data availability statement
TThe raw data supporting the conclusion of this article will be provided by the authors upon a reasonable request.
Ethics statement
The animal study was approved by the Animal Ethics Committee of Mashhad University of Medical Sciences, Mashhad, Iran (IR.MUMS.MEDICAL.REC.1399.426). The study was conducted in accordance with the local legislation and institutional requirements.
Author contributions
AH: conceptualization, formal analysis, investigation, methodology, supervision, writing–original draft, and writing–review and editing. AG: formal analysis, investigation, methodology, writing–original draft, and writing–review and editing. MA: formal analysis, methodology, and writing–original draft. NF: investigation and writing–review and editing. AR: investigation and writing–review and editing. SB-N: investigation and writing–review and editing. AS: conceptualization, investigation, supervision, and writing–review and editing. AE: conceptualization, formal analysis, supervision, writing–original draft, and writing–review and editing.
Funding
The authors declare financial support was received for the research, authorship, and/or publication of this article. This work was supported by the Vice Chancellor for Research and Technology of Mashhad University of Medical Sciences (Grant Number 990600). Open Access funding provided by the Qatar National Library.
Conflict of interest
The authors declare that the research was conducted in the absence of any commercial or financial relationships that could be construed as a potential conflict of interest.
The authors declare that they were editorial board members of Frontiers, at the time of submission. This had no impact on the peer review process and the final decision.
Publisher’s note
All claims expressed in this article are solely those of the authors and do not necessarily represent those of their affiliated organizations, or those of the publisher, the editors, and the reviewers. Any product that may be evaluated in this article, or claim that may be made by its manufacturer, is not guaranteed or endorsed by the publisher.
References
Aebi, H. (1984). Catalase in vitro. Methods Enzymol. 105, 121–126. doi:10.1016/s0076-6879(84)05016-3
Al Dhaheri, Y., Attoub, S., Ramadan, G., Arafat, K., Bajbouj, K., Karuvantevida, N., et al. (2014). Carnosol induces ROS-mediated beclin1-independent autophagy and apoptosis in triple negative breast cancer. PLoS One 9 (10), e109630. Epub 2014/10/10 PubMed PMID: 25299698; PubMed Central PMCID: PMCPMC4192122. doi:10.1371/journal.pone.0109630
Al Disi, S. S., Anwar, M. A., and Eid, A. H. (2015). Anti-hypertensive herbs and their mechanisms of action: Part I. Front. Pharmacol. 6, 323. Epub 2016/02/03 PubMed PMID: 26834637; PubMed Central PMCID: PMCPMC4717468. doi:10.3389/fphar.2015.00323
AlKahlout, A., Fardoun, M., Mesmar, J., Abdallah, R., Badran, A., Nasser, S. A., et al. (2022). Origanum syriacum L. Attenuates the malignant phenotype of MDA-mb231 breast cancer cells. Front. Oncol. 12, 922196. Epub 2022/07/19 PubMed PMID: 35847867; PubMed Central PMCID: PMCPMC9280492. doi:10.3389/fonc.2022.922196
Alsamri, H., Alneyadi, A., Muhammad, K., Ayoub, M. A., Eid, A., and Iratni, R. (2022). Carnosol induces p38-mediated ER stress response and autophagy in human breast cancer cells. Front. Oncol. 12, 911615. Epub 2022/06/18 PubMed PMID: 35712465; PubMed Central PMCID: PMCPMC9194514. doi:10.3389/fonc.2022.911615
Alsamri, H., Athamneh, K., Pintus, G., Eid, A. H., and Iratni, R. (2021b). Pharmacological and antioxidant activities of rhus coriaria L. (Sumac). Antioxidants (Basel) 10 (1), 73. Epub 2021/01/13 PubMed PMID: 33430013; PubMed Central PMCID: PMCPMC7828031. doi:10.3390/antiox10010073
Alsamri, H., El Hasasna, H., Al Dhaheri, Y., Eid, A. H., Attoub, S., and Iratni, R. (2019). Carnosol, a natural polyphenol, inhibits migration, metastasis, and tumor growth of breast cancer via a ROS-dependent proteasome degradation of STAT3. Front. Oncol. 9, 743. Epub 2019/08/29 PubMed PMID: 31456939; PubMed Central PMCID: PMCPMC6698796. doi:10.3389/fonc.2019.00743
Alsamri, H., Hasasna, H. E., Baby, B., Alneyadi, A., Dhaheri, Y. A., Ayoub, M. A., et al. (2021a). Carnosol is a novel inhibitor of p300 acetyltransferase in breast cancer. Front. Oncol. 11, 664403. Epub 2021/06/01 PubMed PMID: 34055630; PubMed Central PMCID: PMCPMC8155611. doi:10.3389/fonc.2021.664403
Ansari, M. A., Iqubal, A., Ekbbal, R., and Haque, S. E. (2019). Effects of nimodipine, vinpocetine and their combination on isoproterenol-induced myocardial infarction in rats. Biomed. Pharmacother. 109, 1372–1380. doi:10.1016/j.biopha.2018.10.199
Anwar, M. A., Al Disi, S. S., and Eid, A. H. (2016). Anti-Hypertensive herbs and their mechanisms of action: Part II. Front. Pharmacol. 7, 50. Epub 2016/03/26 PubMed PMID: 27014064; PubMed Central PMCID: PMCPMC4782109. doi:10.3389/fphar.2016.00050
Anwar, M. A., Samaha, A. A., Baydoun, S., Iratni, R., and Eid, A. H. (2018). Rhus coriaria L. (Sumac) evokes endothelium-dependent vasorelaxation of rat aorta: involvement of the cAMP and cGMP pathways. Front. Pharmacol. 9, 688. Epub 2018/07/14 PubMed PMID: 30002626; PubMed Central PMCID: PMCPMC6031713. doi:10.3389/fphar.2018.00688
Aramouni, K., Assaf, R., Shaito, A., Fardoun, M., Al-Asmakh, M., Sahebkar, A., et al. (2023). Biochemical and cellular basis of oxidative stress: implications for disease onset. J. Cell Physiol. 238 (9), 1951–1963. Epub 2023/07/12 PubMed PMID: 37436042. doi:10.1002/jcp.31071
Arumugam, S., Thandavarayan, R. A., Arozal, W., Sari, F. R., Giridharan, V. V., Soetikno, V., et al. (2012). Quercetin offers cardioprotection against progression of experimental autoimmune myocarditis by suppression of oxidative and endoplasmic reticulum stress via endothelin-1/MAPK signalling. Free Radic. Res. 46 (2), 154–163. Epub 2011/12/08 PubMed PMID: 22145946. doi:10.3109/10715762.2011.647010
Athamneh, K., Hasasna, H. E., Samri, H. A., Attoub, S., Arafat, K., Benhalilou, N., et al. (2017). Rhus coriaria increases protein ubiquitination, proteasomal degradation and triggers non-canonical Beclin-1-independent autophagy and apoptotic cell death in colon cancer cells. Sci. Rep. 7 (1), 11633. Epub 2017/09/16 PubMed PMID: 28912474; PubMed Central PMCID: PMCPMC5599689. doi:10.1038/s41598-017-11202-3
Aydin, S., Ugur, K., Aydin, S., Sahin, İ., and Yardim, M. (2019). Biomarkers in acute myocardial infarction: current perspectives. Vasc. health risk Manag. 15, 1–10. doi:10.2147/VHRM.S166157
Aziz, T. A. (2021a). Cardioprotective effect of quercetin and sitagliptin in doxorubicin-induced cardiac toxicity in rats. Cancer Manag. Res. 13, 2349–2357. Epub 2021/03/20 PubMed PMID: 33737832; PubMed Central PMCID: PMCPMC7965691. doi:10.2147/cmar.S300495
Aziz, T. A. (2021b). Cardioprotective effect of quercetin and sitagliptin in doxorubicin-induced cardiac toxicity in rats. Cancer Manag. Res. 13, 2349–2357. doi:10.2147/CMAR.S300495
Badran, A., Baydoun, E., Samaha, A., Pintus, G., Mesmar, J., Iratni, R., et al. (2019). Marjoram relaxes rat thoracic aorta via a PI3-K/eNOS/cGMP pathway. Biomolecules 9 (6), 227. Epub 2019/06/20PubMed PMID: 31212721; PubMed Central PMCID: PMCPMC6627793. doi:10.3390/biom9060227
Bertinchant, J., Robert, E., Polge, A., Marty-Double, C., Fabbro-Peray, P., Poirey, S., et al. (2000). Comparison of the diagnostic value of cardiac troponin I and T determinations for detecting early myocardial damage and the relationship with histological findings after isoprenaline-induced cardiac injury in rats. Clin. Chim. Acta 298 (1-2), 13–28. doi:10.1016/s0009-8981(00)00223-0
Boroushaki, M. T., Fanoudi, S., Mollazadeh, H., Boroumand-Noughabi, S., and Hosseini, A. (2019). Reno-protective effect of Rheum turkestanicum against gentamicin-induced nephrotoxicity. Iran. J. basic Med. Sci. 22 (3), 328–333. doi:10.22038/ijbms.2019.31552.7597
Chen, J. Y., Hu, R. Y., and Chou, H. C. (2013). Quercetin-induced cardioprotection against doxorubicin cytotoxicity. J. Biomed. Sci. 20 (1), 95. Epub 2013/12/24 PubMed PMID: 24359494; PubMed Central PMCID: PMCPMC3898810. doi:10.1186/1423-0127-20-95
Ćirović, N., Barjaktarevic, A., Ninkovic, M., Bauer, R., Nikles, S., Branković, S., et al. (2021). Biological activities of sanguisorba minor l. extracts ñ in vitro and in vivo evaluations. Acta Poloniae Pharmaceutica-Drug Res. 77, 745–758. doi:10.32383/appdr/127765
Cote, B., Carlson, L. J., Rao, D. A., and Alani, A. W. G. (2015). Combinatorial resveratrol and quercetin polymeric micelles mitigate doxorubicin induced cardiotoxicity in vitro and in vivo. J. Control Release 213, 128–133. Epub 2015/07/15 PubMed PMID: 26160305. doi:10.1016/j.jconrel.2015.06.040
Cristina, T. M. A., Ramona, M. A., Ioana, V. S., and Petru, B. (2021). Antioxidant capacity of sanguisorba officinalis l. and sanguisorba minor scop. Sustain. Dev. 11 (1), 121–133. doi:10.31924/nrsd.v11i1.072
Devika, P. T., and Prince, P. S. M. (2008). (-)-Epigallocatechin gallate (EGCG) prevents isoprenaline-induced cardiac marker enzymes and membrane-bound ATPases. J. Pharm. Pharmacol. 60 (1), 125–133. doi:10.1211/jpp.60.1.0016
Du, Y., Han, J., Zhang, H., Xu, J., Jiang, L., and Ge, W. (2019). Kaempferol prevents against ang II-induced cardiac remodeling through attenuating ang II-induced inflammation and oxidative stress. J. Cardiovasc Pharmacol. 74 (4), 326–335. Epub 2019/07/30 PubMed PMID: 31356553; PubMed Central PMCID: PMCPMC6791499. doi:10.1097/fjc.0000000000000713
El-Gohary, O. A., and Allam, M. M. (2017). Effect of vitamin D on isoprenaline-induced myocardial infarction in rats: possible role of peroxisome proliferator-activated receptor-γ. Can. J. Physiol. Pharmacol. 95 (6), 641–646. doi:10.1139/cjpp-2016-0150
El Hasasna, H., Athamneh, K., Al Samri, H., Karuvantevida, N., Al Dhaheri, Y., Hisaindee, S., et al. (2015). Rhus coriaria induces senescence and autophagic cell death in breast cancer cells through a mechanism involving p38 and ERK1/2 activation. Sci. Rep. 5, 13013. Epub 2015/08/13 PubMed PMID: 26263881; PubMed Central PMCID: PMCPMC4532997. doi:10.1038/srep13013
El Hasasna, H., Saleh, A., Al Samri, H., Athamneh, K., Attoub, S., Arafat, K., et al. (2016). Rhus coriaria suppresses angiogenesis, metastasis and tumor growth of breast cancer through inhibition of STAT3, NFκB and nitric oxide pathways. Sci. Rep. 6, 21144. Epub 2016/02/19 PubMed PMID: 26888313; PubMed Central PMCID: PMCPMC4758048. doi:10.1038/srep21144
Elhemely, M. A., Omar, H. A., Ain-Shoka, A. A., Abd El-Latif, H. A., Abo-youssef, A. M., and El Sherbiny, G. A. (2014). Rosuvastatin and ellagic acid protect against isoproterenol-induced myocardial infarction in hyperlipidemic rats. Beni-Suef Univ. J. Basic Appl. Sci. 3 (4), 239–246. doi:10.1016/j.bjbas.2014.10.010
Fardoun, M., Iratni, R., Dehaini, H., Eid, A., Ghaddar, T., El-Elimat, T., et al. (2019). 7-O-methylpunctatin, a novel homoisoflavonoid, inhibits phenotypic switch of human arteriolar smooth muscle cells. Biomolecules 9 (11), 716. Epub 2019/11/14 PubMed PMID: 31717401; PubMed Central PMCID: PMCPMC6920859. doi:10.3390/biom9110716
Fardoun, M. M., Maaliki, D., Halabi, N., Iratni, R., Bitto, A., Baydoun, E., et al. (2020). Flavonoids in adipose tissue inflammation and atherosclerosis: one arrow, two targets. Clin. Sci. (Lond) 134 (12), 1403–1432. Epub 2020/06/2 PubMed PMID: 32556180. doi:10.1042/CS20200356
Ferenczyova, K., Kalocayova, B., and Bartekova, M. (2020). Potential implications of quercetin and its derivatives in cardioprotection. Int. J. Mol. Sci. 21 (5), 1585. Epub 2020/03/01 PubMed PMID: 32111033; PubMed Central PMCID: PMCPMC7084176. doi:10.3390/ijms21051585
Filipský, T., Zatloukalova, L., Mladěnka, P., and Hrdina, R. (2012). Acute initial haemodynamic changes in a rat isoprenaline model of cardiotoxicity. Hum. Exp. Toxicol. 31 (8), 830–843. doi:10.1177/0960327112438927
Finimundy, T. C., Karkanis, A., Fernandes, Â., Petropoulos, S. A., Calhelha, R., Petrović, J., et al. (2020). Bioactive properties of Sanguisorba minor L. cultivated in central Greece under different fertilization regimes. Food Chem. 327, 127043. doi:10.1016/j.foodchem.2020.127043
Giannouli, M., Karagkiozaki, V., Pappa, F., Moutsios, I., Gravalidis, C., and Logothetidis, S. (2018). Fabrication of quercetin-loaded PLGA nanoparticles via electrohydrodynamic atomization for cardiovascular disease. Mater. Today Proc. 5 (8), 15998–16005. doi:10.1016/j.matpr.2018.05.044
Hesari, M., Mohammadi, P., Khademi, F., Shackebaei, D., Momtaz, S., Moasefi, N., et al. (2021). Current advances in the use of nanophytomedicine therapies for human cardiovascular diseases. Int. J. nanomedicine 16, 3293–3315. doi:10.2147/IJN.S295508
Hosseini, A., Mollazadeh, H., Amiri, M. S., Sadeghnia, H. R., and Ghorbani, A. (2017). Effects of a standardized extract of Rheum turkestanicum Janischew root on diabetic changes in the kidney, liver and heart of streptozotocin-induced diabetic rats. Biomed. Pharmacother. 86, 605–611. doi:10.1016/j.biopha.2016.12.059
Hosseini, A., Safari, M. K., Rajabian, A., Boroumand-Noughabi, S., Eid, A. H., Al Dhaheri, Y., et al. (2022). Cardioprotective effect of rheum turkestanicum against doxorubicin-induced toxicity in rats. Front. Pharmacol. 13, 909079. Epub 2022/06/28 PubMed PMID: 35754479; PubMed Central PMCID: PMCPMC9213811. doi:10.3389/fphar.2022.909079
Izzo, A. A., Teixeira, M., Alexander, S. P., Cirino, G., Docherty, J. R., George, C. H., et al. (2020). A practical guide for transparent reporting of research on natural products in the British Journal of Pharmacology: reproducibility of natural product research. Hoboken, New Jersey, United States: Wiley Online Library, 2169–2178.
Jung, D.-W., Lee, O.-H., and Kang, I.-J. (2016). Sanguisorba officinalis L. extracts exert antiobesity effects in 3T3-L1 adipocytes and C57BL/6J mice fed high-fat diets. J. Med. Food 19 (8), 768–779. doi:10.1089/jmf.2016.3704
Kamisah, Y., Jalil, J., Yunos, N. M., and Zainalabidin, S. (2023). Cardioprotective properties of kaempferol: a review. Plants (Basel) 12 (11), 2096. Epub 2023/06/10 PubMed PMID: 37299076; PubMed Central PMCID: PMCPMC10255479. doi:10.3390/plants12112096
Kannan, M. M., and Quine, S. D. (2011). Ellagic acid ameliorates isoproterenol induced oxidative stress: evidence from electrocardiological, biochemical and histological study. Eur. J. Pharmacol. 659 (1), 45–52. Epub 2011/03/10 PubMed PMID: 21385579. doi:10.1016/j.ejphar.2011.02.037
Karkanis, A. C., Fernandes, Â., Vaz, J., Petropoulos, S., Georgiou, E., Ciric, A., et al. (2019). Chemical composition and bioactive properties of Sanguisorba minor Scop. under Mediterranean growing conditions. Food Funct. 10 (3), 1340–1351. Epub 2019/02/15 PubMed PMID: 30761392. doi:10.1039/c8fo02601g
Klevanova, V., and Trzhetsynskiy, S. (2015). Antidiabetic activity of Poterium sanguisorba L. extract on streptozotocinnicotinamide induced diabetic rat. J. Chem. Pharm. Res.,
Kumar, M., Kasala, E. R., Bodduluru, L. N., Kumar, V., and Lahkar, M. (2017). Molecular and biochemical evidence on the protective effects of quercetin in isoproterenol-induced acute myocardial injury in rats. J. Biochem. Mol. Toxicol. 31 (1), 1–8. Epub 2016/08/17 PubMed PMID: 27527349. doi:10.1002/jbt.21832
Lewandrowski, K., Chen, A., and Januzzi, J. (2002). Cardiac markers for myocardial infarction: a brief review. Pathol. Patterns Rev. 118 (Suppl. l_1), S93–S99. doi:10.1309/3EK7-YVV9-228C-E1XT
Li, Y., Yao, J., Han, C., Yang, J., Chaudhry, M. T., Wang, S., et al. (2016). Quercetin, inflammation and immunity. Nutrients 8 (3), 167. Epub 2016/03/22 PubMed PMID: 26999194; PubMed Central PMCID: PMCPMC4808895. doi:10.3390/nu8030167
Maaliki, D., Shaito, A. A., Pintus, G., El-Yazbi, A., and Eid, A. H. (2019). Flavonoids in hypertension: a brief review of the underlying mechanisms. Curr. Opin. Pharmacol. 45, 57–65. Epub 2019/05/19 PubMed PMID: 31102958. doi:10.1016/j.coph.2019.04.014
Madesh, M., and Balasubramanian, K. (1998). Microtiter plate assay for superoxide dismutase using MTT reduction by superoxide. Indian J. Biochem. Biophys. 35 (3), 184–188.
Manjula, T., Geetha, A., Ramesh, T., and Devi, C. S. (1992). Reversal of changes of myocardial lipids by chronic administration of aspirin in isoproterenol-induced myocardial damage in rats. Indian J. Physiol. Pharmacol. 36, 47–50.
Mari Kannan, M., and Darlin Quine, S. (2011). Pharmacodynamics of ellagic acid on cardiac troponin-T, lyosomal enzymes and membrane bound ATPases: mechanistic clues from biochemical, cytokine and in vitro studies. Chem. Biol. Interact. 193 (2), 154–161. doi:10.1016/j.cbi.2011.06.005
McCord, J. M., and Fridovich, I. (1969). Superoxide dismutase: an enzymic function for erythrocuprein (hemocuprein). J. Biol. Chem. 244 (22), 6049–6055. doi:10.1016/s0021-9258(18)63504-5
Mesmar, J., Fardoun, M. M., Abdallah, R., Al Dhaheri, Y., Yassine, H. M., Iratni, R., et al. (2021). Ziziphus nummularia attenuates the malignant phenotype of human pancreatic cancer cells: role of ROS. Molecules 26 (14), 4295. Epub 2021/07/25PubMed PMID: 34299570; PubMed Central PMCID: PMCPMC8307183. doi:10.3390/molecules26144295
Norouzi, F., Hosseini, M., Abareshi, A., Beheshti, F., Khazaei, M., Shafei, M. N., et al. (2019). Memory enhancing effect of Nigella Sativa hydro-alcoholic extract on lipopolysaccharide-induced memory impairment in rats. Drug Chem. Toxicol. 42 (3), 270–279. doi:10.1080/01480545.2018.1447578
Panda, S., and Kar, A. (2015). Combined effects of vincristine and quercetin in reducing isoproterenol-induced cardiac necrosis in rats. Cardiovasc Toxicol. 15 (4), 291–299. doi:10.1007/s12012-014-9291-x
Paritha, I. A., and Shyamala, D. C. (1997). Effect of alpha-tocopherol on isoproterenol-induced changes in lipid and lipoprotein profile in rats. Indian J. Pharmacol. 29 (6), 399.
Patel, V., Upaganlawar, A., Zalawadia, R., and Balaraman, R. (2010). Cardioprotective effect of melatonin against isoproterenol induced myocardial infarction in rats: a biochemical, electrocardiographic and histoarchitectural evaluation. Eur. J. Pharmacol. 644 (1-3), 160–168. doi:10.1016/j.ejphar.2010.06.065
Preus, M., Bhargava, A. S., El Rahman Khater, A., and Gunzel, P. (1988). Diagnostic value of serum creatine kinase and lactate dehydrogenase isoenzyme determinations for monitoring early cardiac damage in rats. Toxicol. Lett. 42 (2), 225–233. doi:10.1016/0378-4274(88)90081-1
Qi, Y., Ying, Y., Zou, J., Fang, Q., Yuan, X., Cao, Y., et al. (2020). Kaempferol attenuated cisplatin-induced cardiac injury via inhibiting STING/NF-κB-mediated inflammation. Am. J. Transl. Res. 12 (12), 8007–8018. Epub 2021/01/14. PubMed PMID: 33437376; PubMed Central PMCID: PMCPMC7791507.
Rao, P. R., and Viswanath, R. K. (2007). Cardioprotective activity of silymarin in ischemia-reperfusion-induced myocardial infarction in albino rats. Exp. Clin. Cardiol. 12 (4), 179–187. Epub 2008/07/25. PubMed PMID: 18651002; PubMed Central PMCID: PMCPMC2359609.
Safarpour, S., Pirzadeh, M., Ebrahimpour, A., Shirafkan, F., Madani, F., Hosseini, M., et al. (2022). Protective effect of kaempferol and its nanoparticles on 5-fluorouracil-induced cardiotoxicity in rats. Biomed. Res. Int. 2022, 2273000. Epub 2022/02/25 PubMed PMID: 35198633; PubMed Central PMCID: PMCPMC8858719. doi:10.1155/2022/2273000
Salama, M., Ezzat, S. M., and Salem, M. (2020). Natural products for the management of cardiovascular diseases. Pharmacy 489.
Samaha, A. A., Fawaz, M., Salami, A., Baydoun, S., and Eid, A. H. (2019). Antihypertensive indigenous Lebanese plants: ethnopharmacology and a clinical trial. Biomolecules 9 (7), 292. Epub 2019/07/25 PubMed PMID: 31330767; PubMed Central PMCID: PMCPMC6681041. doi:10.3390/biom9070292
Saravanan, G., and Prakash, J. (2004). Effect of garlic (Allium sativum) on lipid peroxidation in experimental myocardial infarction in rats. J. Ethnopharmacol. 94 (1), 155–158. doi:10.1016/j.jep.2004.04.029
Shaito, A., Aramouni, K., Assaf, R., Parenti, A., Orekhov, A., Yazbi, A. E., et al. (2022). Oxidative stress-induced endothelial dysfunction in cardiovascular diseases. Front. Biosci. (Landmark Ed. 27 (3), 105. Epub 2022/03/3 PubMed PMID: 35345337. doi:10.31083/j.fbl2703105
Shen, Z., Geng, Q., Huang, H., Yao, H., Du, T., Chen, L., et al. (2019). Antioxidative and cardioprotective effects of Schisandra chinensis bee pollen extract on isoprenaline-induced myocardial infarction in rats. Molecules 24 (6), 1090. doi:10.3390/molecules24061090
Shiny, K., Kumar, S. H. S., Farvin, K. S., Anandan, R., and Devadasan, K. (2005). Protective effect of taurine on myocardial antioxidant status in isoprenaline-induced myocardial infarction in rats. J. Pharm. Pharmacol. 57 (10), 1313–1317. doi:10.1211/jpp.57.10.0010
Shouk, R., Abdou, A., Shetty, K., Sarkar, D., and Eid, A. H. (2014). Mechanisms underlying the antihypertensive effects of garlic bioactives. Nutr. Res. 34 (2), 106–115. Epub 2014/01/28 PubMed PMID: 24461311. doi:10.1016/j.nutres.2013.12.005
Slika, H., Mansour, H., Wehbe, N., Nasser, S. A., Iratni, R., Nasrallah, G., et al. (2022). Therapeutic potential of flavonoids in cancer: ROS-mediated mechanisms. Biomed. Pharmacother. 146, 112442. Epub 2022/01/23 PubMed PMID: 35062053. doi:10.1016/j.biopha.2021.112442
Strålfors, P., Björgell, P., and Belfrage, P. (1984). Hormonal regulation of hormone-sensitive lipase in intact adipocytes: identification of phosphorylated sites and effects on the phosphorylation by lipolytic hormones and insulin. Proc. Natl. Acad. Sci. 81 (11), 3317–3321. doi:10.1073/pnas.81.11.3317
Suchal, K., Malik, S., Gamad, N., Malhotra, R. K., Goyal, S. N., Bhatia, J., et al. (2016). Kampeferol protects against oxidative stress and apoptotic damage in experimental model of isoproterenol-induced cardiac toxicity in rats. Phytomedicine 23 (12), 1401–1408. doi:10.1016/j.phymed.2016.07.015
Surekha, R., Srikanth, B., Jharna, P., Ramachandra, R., Dayasagar, R., and Jyothy, A. (2007). Oxidative stress and total antioxidant status in myocardial infarction. Singap. Med. J. 48 (2), 137–142.
Thang, B. Q., Sakamoto, H., Matsushita, S., and Hiramatsu, Y. (2018). “A convenient mouse model of myocardial infarction for developing countries,” in 6th International Conference on the Development of Biomedical Engineering in Vietnam (BME6), Ho Chi Minh, Vietnam, June, 2018.
Thuan, D. T. B., Zayed, H., Eid, A. H., Abou-Saleh, H., Nasrallah, G. K., Mangoni, A. A., et al. (2018). A potential link between oxidative stress and endothelial-to-mesenchymal transition in systemic sclerosis. Front. Immunol. 9, 1985. Epub 2018/10/05 PubMed PMID: 30283435; PubMed Central PMCID: PMCPMC6156139. doi:10.3389/fimmu.2018.01985
Tiwari, R., Mohan, M., Kasture, S., Maxia, A., and Ballero, M. (2009). Cardioprotective potential of myricetin in isoproterenol-induced myocardial infarction in Wistar rats. Phytotherapy Res. Int. J. Devoted Pharmacol. Toxicol. Eval. Nat. Prod. Deriv. 23 (10), 1361–1366. doi:10.1002/ptr.2688
Tocai Moţoc, A. C., Kokeric, T., Tripon, S., Barbu-Tudoran, L., Barjaktarevic, A., Cupara, S., et al. (2023). Sanguisorba minor scop.: an overview of its phytochemistry and biological effects. Plants (Basel) 12 (11), 2128. Epub 2023/06/10 PubMed PMID: 37299107; PubMed Central PMCID: PMCPMC10255321. doi:10.3390/plants12112128
Todd, G. L., Cullan, G. E., and Cullan, G. M. (1980). Isoproterenol-induced myocardial necrosis and membrane permeability alterations in the isolated perfused rabbit heart. Exp. Mol. Pathol. 33 (1), 43–54. doi:10.1016/0014-4800(80)90006-4
Upaganlawar, A., and Balaraman, R. (2011). Cardioprotective effects of Lagenaria siceraria fruit juice on isoproterenol-induced myocardial infarction in wistar rats: a biochemical and histoarchitecture study. J. Young Pharm. 3 (4), 297–303. doi:10.4103/0975-1483.90241
Upaganlawar, A., Gandhi, H., and Balaraman, R. (2011). Isoproterenol induced myocardial infarction: protective role of natural products. J. Pharmacol. Toxicol. 6 (1), 1–17. doi:10.3923/jpt.2011.1.17
Vishwakarma, A., Singh, T. U., Rungsung, S., Kumar, T., Kandasamy, A., Parida, S., et al. (2018a). Effect of kaempferol pretreatment on myocardial injury in rats. Cardiovasc Toxicol. 18 (4), 312–328. doi:10.1007/s12012-018-9443-5
Vishwakarma, A., Singh, T. U., Rungsung, S., Kumar, T., Kandasamy, A., Parida, S., et al. (2018b). Effect of kaempferol pretreatment on myocardial injury in rats. Cardiovasc Toxicol. 18 (4), 312–328. Epub 2018/01/22 PubMed PMID: 29353381. doi:10.1007/s12012-018-9443-5
Wang, Y., Zhang, Z. Z., Wu, Y., Ke, J. J., He, X. H., and Wang, Y. L. (2013). Quercetin postconditioning attenuates myocardial ischemia/reperfusion injury in rats through the PI3K/Akt pathway. Braz J. Med. Biol. Res. 46 (10), 861–867. Epub 2013/09/27 PubMed PMID: 24068165; PubMed Central PMCID: PMCPMC3854307. doi:10.1590/1414-431x20133036
Warpe, V. S., Mali, V. R., Arulmozhi, S., Bodhankar, S. L., and Mahadik, K. R. (2015). Cardioprotective effect of ellagic acid on doxorubicin induced cardiotoxicity in wistar rats. J. acute Med. 5 (1), 1–8. doi:10.1016/j.jacme.2015.02.003
Weil, B. R., Young, R. F., Shen, X., Suzuki, G., Qu, J., Malhotra, S., et al. (2017). Brief myocardial ischemia produces cardiac troponin I release and focal myocyte apoptosis in the absence of pathological infarction in swine. Basic Transl. Sci. 2 (2), 105–114. doi:10.1016/j.jacbts.2017.01.006
Xiao, J., Sun, G. B., Sun, B., Wu, Y., He, L., Wang, X., et al. (2012). Kaempferol protects against doxorubicin-induced cardiotoxicity in vivo and in vitro. Toxicology 292 (1), 53–62. Epub 2011/12/14 PubMed PMID: 22155320. doi:10.1016/j.tox.2011.11.018
Yaseen, A. E.-R. A., Shaban, M. I., El-Odemi, M. H., El-Fiky, S. R., and Shebl, D. Z. M. (2017). Potential protective effects of trimetazidine and quercetin on isoprenaline-induced myocardial infarction in rats. Menoufia Med. J. 30 (4), 1110. doi:10.4103/mmj.mmj_183_17
Zaafan, M. A., Zaki, H. F., El-Brairy, A. I., and Kenawy, S. A. (2013). Protective effects of atorvastatin and quercetin on isoprenaline-induced myocardial infarction in rats. Bull. Fac. Pharm. 51 (1), 35–41. doi:10.1016/j.bfopcu.2013.03.001
Zhang, J., Knapton, A., Lipshultz, S. E., Weaver, J. L., and Herman, E. H. (2008). Isoproterenol-induced cardiotoxicity in sprague-dawley rats: correlation of reversible and irreversible myocardial injury with release of cardiac troponin T and roles of iNOS in myocardial injury. Toxicol. Pathol. 36 (2), 277–278. doi:10.1177/0192623307313010
Zhao, Z., He, X., Zhang, Q., Wei, X., Huang, L., Fang, J. C., et al. (2017). Traditional uses, chemical constituents and biological activities of plants from the genus sanguisorba L. Am. J. Chin. Med. 45 (02), 199–224. doi:10.1142/S0192415X17500136
Keywords: myocardial infarction, isoprenaline, oxidative stress, cardiotoxicity, herbal medicine, superoxide dismutase
Citation: Hosseini A, Ghorbani A, Alavi MS, Forouhi N, Rajabian A, Boroumand-Noughabi S, Sahebkar A and Eid AH (2023) Cardioprotective effect of Sanguisorba minor against isoprenaline-induced myocardial infarction in rats. Front. Pharmacol. 14:1305816. doi: 10.3389/fphar.2023.1305816
Received: 02 October 2023; Accepted: 27 November 2023;
Published: 21 December 2023.
Edited by:
Syed Shams ul Hassan, Chinese Academy of Sciences, ChinaReviewed by:
Abdelhabib Semlali, Laval University, CanadaNarasimham L. Parinandi, The Ohio State University, United States
Bruno Moukette, Indiana University—Purdue University Indianapolis, United States
Muhammad Majid, Hamdard University, Pakistan
Copyright © 2023 Hosseini, Ghorbani, Alavi, Forouhi, Rajabian, Boroumand-Noughabi, Sahebkar and Eid. This is an open-access article distributed under the terms of the Creative Commons Attribution License (CC BY). The use, distribution or reproduction in other forums is permitted, provided the original author(s) and the copyright owner(s) are credited and that the original publication in this journal is cited, in accordance with accepted academic practice. No use, distribution or reproduction is permitted which does not comply with these terms.
*Correspondence: Amirhossein Sahebkar, amir_saheb2000@yahoo.com; Ali H. Eid, ali.eid@qu.edu.qa