- 1Botany and Microbiology Department, Faculty of Science, Benha University, Benha, Egypt
- 2Department of Plant Agriculture, University of Guelph, Guelph, ON, Canada
- 3Department of Genetics, Faculty of Agriculture, Menoufia University, Shebeen El-Kom, Egypt
- 4Department of Biology, College of Science, University of Hafr Al Batin, Hafar Al Batin, Saudi Arabia
Plant-associated microbes play crucial roles in plant health and promote growth under stress. Tomato (Solanum lycopersicum) is one of the strategic crops grown throughout Egypt and is a widely grown vegetable worldwide. However, plant disease severely affects tomato production. The post-harvest disease (Fusarium wilt disease) affects food security globally, especially in the tomato fields. Thus, an alternative effective and economical biological treatment to the disease was recently established using Trichoderma asperellum. However, the role of rhizosphere microbiota in the resistance of tomato plants against soil-borne Fusarium wilt disease (FWD) remains unclear. In the current study, a dual culture assay of T. asperellum against various phytopathogens (e.g., Fusarium oxysporum, F. solani, Alternaria alternata, Rhizoctonia solani, and F. graminearum) was performed in vitro. Interestingly, T. asperellum exhibited the highest mycelial inhibition rate (53.24%) against F. oxysporum. In addition, 30% free cell filtrate of T. asperellum inhibited F. oxysporum by 59.39%. Various underlying mechanisms were studied to explore the antifungal activity against F. oxysporum, such as chitinase activity, analysis of bioactive compounds by gas chromatography–mass spectrometry (GC–MS), and assessment of fungal secondary metabolites against F. oxysporum mycotoxins in tomato fruits. Additionally, the plant growth-promoting traits of T. asperellum were studied (e.g., IAA production, Phosphate solubilization), and the impact on tomato seeds germination. Scanning electron microscopy, plant root sections, and confocal microscopy were used to show the mobility of the fungal endophyte activity to promote tomato root growth compared with untreated tomato root. T. asperellum enhanced the growth of tomato seeds and controlled the wilt disease caused by the phytopathogen F. oxysporum by enhancing the number of leaves as well as shoot and root length (cm) and fresh and dry weights (g). Furthermore, Trichoderma extract protects tomato fruits from post-harvest infection by F. oxysporum. Taking together, T. asperellum represents a safe and effective controlling agent against Fusarium infection of tomato plants.
Introduction
Tomato (Solanum lycopersicon L.) is one of the most essential and widespread horticultural crops worldwide (Pritesh and Subramanian, 2011; Bawa, 2016), and it is a rich source of vitamins A and C (Pramanik and Mohapatra, 2017; Boccia et al., 2019). Egypt is a top-five tomato producer, accounting for 7.2 to 8.6 million tons annually (Nakai, 2018). However, there is a significant concern regarding food security, as demographic projections indicate that the global population will rise to 9.5 billion by 2050 (Attia et al., 2022). Food security and safety are increasingly threatened as the human population continues to expand due to soil-borne fungal pathogens emerging worldwide. In addition, such pathogens can reduce crop productivity in greenhouse and field conditions (e.g., vegetable crops), consequently causing severe losses to global food security (Almeida et al., 2019). Fungal diseases cause over 1.6 million deaths annually, and over one billion people suffer from severe fungal infections. Moreover, fungal pathogens are considered the highest threat to plant-host species, representing the main reason for reducing plant growth (approximately 65%) (Zhang and Ma, 2017; Almeida et al., 2019; Hewedy et al., 2020a,b). In addition, toxigenic fungi are the most important plant pathogens, causing diseases such as Fusarium oxysporum species complex (FOSC) (Zhang and Ma, 2017; Hewedy et al., 2020a,b), Rhizoctonia solani (Ghazala et al., 2022), Fusarium solani (Saengchan et al., 2022), and Fusarium graminearum (de Chaves et al., 2022). The plant root is the key site for interacting with the host plant, microbial pathogens, and the rhizosphere microbiome (Zhalnina et al., 2018). Furthermore, plants have become more susceptible to disease due to continuous exposure to stress and climatic changes worldwide (Attia et al., 2022). Tomato plants are affected by numerous infections caused by many different agents, including fungus, bacteria, viruses, and physiological disorders, responsible for symptoms including fruit spots, rots, wilts, and leaf spots/blights (Jones et al., 2014; Elshafie et al., 2017). F. oxysporum f. sp. lycopersici (Fol) is a potent fungal pathogen infects tomato crops by penetrating the roots. This pathogen causes yellowing of lower leaves, browning of vascular tissues, and wilting symptoms in tomato seedlings above the soil line with substantial yield losses (Borrero et al., 2012; Li et al., 2018). Moreover, infection of tomato plants is achieved by spore germination or mycelium, leading to higher plant transpiration and lower nutrient translocation, causing wilting crown and root rot and, eventually, death of the plant (Akbar et al., 2018; Manikandan et al., 2018). Fusarium oxysporum is a widely distributed and phylogenetically diverse species known as a mycotoxin producer (Irzykowska et al., 2012) and is ranked fifth out of the 10 most lethal (death-causing) plant pathogens. Two main formae speciales are F. oxysporumf. sp. lycopersici (FOL) and F. oxysporum f. sp. radicis-lycopersici (FORL) (Ribeiro et al., 2022). FOL is responsible for Fusarium wilt, and FORL causes Fusarium crown and root rot, among the most studied plant diseases. In Egypt, the damage to tomato crop production due to F. oxysporum wilt occurred in 67% of the total planted area (Selim and El-Gammal, 2015). In addition, some fungal species produce mycotoxins (e.g., aflatoxin) on plants which negatively affects the post-harvest treatments and helps to spread the causal agent between the next generation of seeds (Marshall et al., 2020). Thus, various methods, such as chemicals and pesticides, are employed to control and alleviate plant diseases (Ristaino et al., 2021). However, using fungicides to control Fusarium wilt is ineffective because fungal conidia stay viable for a long time, and pesticide residues are harmful to human health (Attia et al., 2022). Therefore, new control strategies must be developed with the aim of economic and environmental sustainability in plant and crop protection (Hernández-Aparicio et al., 2021). Biological control is a desirable alternative method to control the Fusarium wilt infection by using antagonistic nonpathogenic microorganisms to minimize the harmful effects in numerous crops (Glick, 2012). Chemical fungicides and soil fumigation have been widely used for controlling FWD disease. Trichoderma strains are vital anti-pathogen biocontrol agents (Pal and Gardener, 2006; Contreras-Cornejo et al., 2016; Zhang et al., 2022). This genus is a ubiquitous filamentous fungi that grow in the rhizosphere and colonizes plant roots as an opportunistic, avirulent plant symbiont (Harman, 2006). As Trichoderma species is a known aggressive wide range of soil fungal pathogens worldwide to suppress soil fungal diseases and plant pathogen invasion. They are frequently applied as biofungicides against pathogens such as Botrytis cinerea, Fusarium spp., Pythium spp., Rhizoctonia solani, and Sclerotium rolfsii on crops of economic importance (Mohiddin et al., 2010; Olowe et al., 2022; Sharma et al., 2022). Trichoderma fungi indirectly exert their biological control machinery toward fungal pathogens by competition for nutrients and space, antibiosis, production of growth-promoting substances (e.g., IAA, nutrient uptake) (Lei and Zhang, 2015; França et al., 2017) or by secretion of bioactive metabolites, some cell wall-degrading enzymes (CWDEs), such as chitinases and β-1,3-glucanases and mycoparasitism (Elshahawy and El-Mohamedy, 2019; Sallam et al., 2019). Mycoparasitism is the most efficient antifungal mechanism of Trichoderma spp. (e.g., T. virens, T. harzianum) via recognizing the pathogen and growing alongside the pathogen hyphae, then dissolution and death of the pathogen (Benítez et al., 2004; Sharon et al., 2007; Hewedy et al., 2020a,b; Contreras-Cornejo et al., 2021; Taylor et al., 2021; Mukherjee et al., 2022). Various factors such as native environmental habitats, strains, pathogen species, and laboratory findings for testing their antifungal activities affect the Trichoderma biocontrol machinery. Trichoderma asperellum is a promising strain based on in vitro assays against plant fungal phytopathogens. For example, T. asperellum GDFS1009 has been shown tosuppress F. oxysporum f. sp. cucumerinum (Fusarium wilt of cucumber) and Fusarium graminearum infections (Wu et al., 2017; Ketta and Hewedy, 2021), with 98 and 91% inhibition of colony radial growth, respectively, to cope with biotic stresses (Kamaruzzaman et al., 2021). In this context, the discovery of a sustainable solution is the main aim of this study to select and test a potent fungal strain to reduce the negative influence of fungal pathogenicity with the aim of controlling plant disease as well as improving plant growth and plant health to sustain food production. Thus, in this study, we sought to isolate T. asperellum from the rhizosphere of garlic (Allium sativum), identified using the ITS gene, test the antifungal activities against different fungal pathogens, and focus on the causal agent that causes FWD. Moreover, exploring the antagonistic mechanisms such as chitinase activity and bioactive secondary metabolites, as well as the role of T. asperellum for tomato growth promotion (e.g., IAA, P solubilization). Moreover, we present a new trend regarding post-harvest applications using fungal secondary metabolites against F. oxysporum mycotoxins to achieve sustainable food production in an eco-friendly environment. Finally, we used confocal microscopy as a throughput technique to explore the mobility of Trichoderma in the tomato roots.
Materials and methods
Phytopathogens and antagonistic strain
Five phytopathogenic fungi (F. oxysporum, F. solani, F. graminearum, Alternaria alternata, and Rhizoctonia solani) were obtained from Agriculture Research Center, Giza, Egypt. Trichoderma isolate (Biocontrol agent) was isolated from the garlic (Allium sativum) rhizosphere soil by serial dilution method. Briefly, the rhizospheric soil sample (10 g) was suspended in 90 mL of sterile distilled water, and the mixture was shaken at (200 rpm) for 30 min. Next, the soil suspension was serially diluted up to (10−3) dilution, then 100 μl was spread on Potato Dextrose Agar (PDA) plates supplemented with chloramphenicol (50 mg/L) and streptomycin (15 mg/L) to suppress bacterial growth (Woldeamanuale, 2017). Subsequently, the inoculated plates were incubated at 28°C for 5–7 days. At the end of the incubation period, the fungal isolate was purified, kept on slants at 4°C, and subcultured every 4 weeks (López Errasquín and Vázquez, 2003). The pure culture of the fungal isolate was characterized using morphological and microscopical characteristics (Harman and Kubicek, 2002) and confirmed by molecular identification.
Molecular identification
Genomic DNA was extracted with the QIAamp DNeasy Plant Mini kit according to the manufacturer’s instructions. Amplification of the fungal ITS region was carried out with forward ITS1 (5′ TCC-GTA-GGT-GAA-CCT-GCG-G 3′) and reverse ITS4 (5′TCC-TCC-GCT-TAT-TGA-TAT-GC 3′) primers (White et al., 1990). The polymerase chain reaction program was performed as previously described (Hewedy et al., 2020c). The homology of the ITS rDNA sequence of the isolate was analyzed using the BLAST program from the GenBank database.1
In vitro antagonistic activity of Trichoderma asperellum against phytopathogenic fungi
The antagonistic activity of T. asperellum against fungal pathogens was evaluated by dual culture assay (Awad et al., 2018). One mycelial disk of the pathogen (6 mm diameter) was deposited on one side of the Petri plate, and another disk of (antagonist) was deposited equidistantly on the other side. PDA plates containing only disks from the pathogen mycelium were used as controls. All the experiments were conducted in triplicates. After 7 days of incubation at 28°C, the radial growth of the phytopathogens in the control and treatment plates was measured, and the percentage of inhibition of radial mycelial growth (PIRG) was calculated using the following equation:
where R1 = radial growth of the phytopathogen in the control plate; R2 = radial growth in the presence of an antagonist (Fishal et al., 2022).
Effect of Trichoderma asperellum culture filtrates on Fusarium oxysporum
Based on the dual culture assay results, the maximum growth inhibition was recorded against F. oxysporum. Thus, different concentrations of the culture of T. asperellum filtrate were tested against F. oxysporum. T. asperellum was inoculated in 50 mL of potato dextrose broth and incubated at 28°C for 14 days on a rotary shaker at 150 rpm. Cell-free supernatant was obtained by filtration through Whatman filter paper No. 1. The filtrate was sterilized using a 0.2 μm pore biological membrane filter (Jangir et al., 2019). Each sterilized filtrate was mixed into a PDA medium to obtain 5, 15, and 30% (v/v). Finally, 20 mL of the medium was amended with different filtrate concentrations and poured into 90 mm Petri plates. The secondary metabolites in the filtrate were tested for their efficacy against the test pathogens. The test pathogen was centrally inoculated with an individual equal disk (5 mm) of seven-day-old culture. PDA plates inoculated with pathogens without culture filtrates served as a control. Three replicates were maintained for each treatment and incubated at 28°C. The percentage inhibition of mycelial growth was calculated as mentioned above.
Scanning electron microscopy
The confrontation of hyphal interaction between F. oxysporum and T. asperellum was also examined by SEM. Briefly, a mycelial disk (5 mm) of both microbes was cultured on PDA for 4–5 days of incubation. The plate cultures were examined under a light microscope to check the early touch stage. The contact interactions were labeled, and 1 cm agar blocks were removed for SEM preparation, fixed with osmium tetroxide, and then dehydrated using a serial dilution of the ethyl alcohol and acetone. A critical point drier (Tousimis Autosamdri–815 Coater) was then used to dry the processed samples with gold using a sputter coater. Then, the mycoparsatism and the hyphae interactions were examined by SEM (JEOL JSM 6510 lv). The microscope was operated at 30 KV at EM Unit, Mansoura University, Egypt. Three replicates were included for each sample (Su et al., 2010).
Assessment of plant growth-promoting and antifungal properties of Trichoderma asperellum
Indole-3- acetic acid
Trichoderma asperellum was grown on potato dextrose broth (PDB) supplemented with L-Tryptophan (0.1 g/L) for 4–5 days at 28 ± 2°C on a rotary shaker at 150 rpm (Nandini et al., 2021). Next, both mycelium and debris were separated by filtration and centrifugation, respectively. Briefly, 1 mL of the culture filtrate and 4 mL of Salkowski reagent (1 mL of 0.5 M ferric chloride in 50 mL of 35% perchloric acid) were mixed and kept for 30 min in the dark for color change. The generation of pink indicated the production of IAA. The optical density was detected and measured at 530 nm using a spectrophotometer (JENWAY 6315). A standard curve of IAA concentrations was designed to evaluate the corresponding concentration of IAA-released T. asperellum in the bioassay media.
Phosphate solubilization efficacy of Trichoderma asperellum
A plug of T.asperellum (5 mm) was grown on a modified NBRIP medium (Nautiyal, 1999) supplemented by Bromophenol blue as an indicator for 7 days at 28°C. The medium contained the following composition (gL−1: 10.0 glucose), 0.5MgCL2.6H2O, 0.25MgSO4.7H2O, 0.2KCL, 0.1 (NH4)2SO4 and 15.0 agar, with the addition of 50 mL of K2HPO4 (10% w/v) and 100 mL of CaCL2 (10% w/v) to precipitate insoluble calcium phosphate (CaHPO4). The pH of the medium was adjusted to 7.0. After incubation, a yellow halo zone around the fungal colony indicated phosphate solubilizing ability. The solubilization zone and colony diameters were measured and calculated using the equation below to indicate the solubilization efficiency (Pande et al., 2017; Aloisio et al., 2019).
SE = (Solubilization diameter)/(Growth diameter) ×100.
Chitinase activity of Trichoderma asperellum
Chitinase detection medium with ingredients/per liter: 0.3 g of MgSO4.7H2O, 3.0 g (NH4)2SO4, 2.0 g of KH2PO4, 1.0 g of citric acid monohydrate, 15 g of agar, 200 μl of Tween-80, 4.5 g of colloidal chitin and 0.15 g of bromocresol purple pH (4.7), was prepared and autoclaved at 121°C for 15 min. Then, the plates were inoculated with a fresh culture plug of T. asperellum, incubated at 25 ± 2°C for 7 days, and were observed for colored zone formation (Agrawal and Kotasthane, 2012).
Trichoderma asperellum promotes tomato plant growth
Inoculum preparation
Seven-day-old pure culture of T. asperellum was used. After cultivation on PDA slants and incubation at 28°C, sterile water was poured into the slants, and the spores were scraped with a sterile glass rod. The spore suspension was filtered and put into tubes, and the spore concentration of T. asperellum was adjusted at 1 × 106 spore/mL (Scudeletti et al., 2021; Natsiopoulos et al., 2022).
Effect of Trichoderma asperellum on tomato seeds germination and growth promotion
Tomato seeds of (Hybrid Madera F1) were used in all experiments. The tomato seeds were surface sterilized with 2.5% (v/v) sodium hypochlorite for 10 min and rinsed three times with distilled water before seed inoculation. Afterward, the seeds were immersed for 24 h in fungal suspensions prepared at 1.0 ×106 spore mL−1. The control treatment consisted of seeds immersed only in sterile distilled water for the same period. The seeds were deposited in Petri dishes containing autoclaved tissue papers moistened with sterile water (16 seeds per dish) that were sealed with parafilm to prevent evaporation and then incubated at 28°C for 7 days (Kipngeno et al., 2015; Barroso et al., 2019). Each treatment was replicated three times. The germination rate (%) was estimated by counting the number of seeds germinating after one week of cultivation.
Three sterilized tomato seeds were transferred to one side of the plate prepared with water agar containing 0.2% (w/v) glucose (Yoo et al., 2018). The other side contained a T. asperellum disk (5 mm) grown on PDA. Plates without inoculum were considered a control, and the plates were incubated for 3–4 days in darkness at 28°C. After the incubation period, the germination percentage (GP) was evaluated according to the following equation:
where: n = number of germinated seeds; N = total number of seeds.
Next, treated and untreated (control) seeds were sown in pots (8 cm) in diameter containing a mixture of sterilized peat moss and soil (1:2 w/w) at 28 g/pot, with a density of five seeds per pot. Pots were maintained under controlled conditions in the growth chamber (22 ± 3°C). Experiments were performed using a completely randomized design (CRD) with two treatments and three replicates. The pots were rotated three times a week to ensure uniform growth conditions in the growth chamber. After 21 days, plant samples were taken to measure the shoot height (cm), the root length (cm), and plant biomass. The seedling vigor index (VI) was calculated as described by Buriro et al. (2011).
Confocal microscopic assay
The interaction between T. asperellum strain and the tomato-root system during the early stages of root colonization was examined by confocal microscopy. SYTOX Green (Thermo Fisher Scientific-United States) was used for Trichoderma staining. Briefly, tomato roots were carefully removed from the sterilized soil and gently swirled 4–5 times in autoclaved water to wash the clay particles. Then, the primary root was stained using 10 μM propidium iodide (PI) for 10 min to label the plant cell wall and washed by ddH2O 4–5 times. Subsequently, the root was placed on a glass slide to facilitate the visualization of the localization of T. asperellum (green) in the tomato root (red) surface as a plant growth promoter (Chacón et al., 2007).
Estimation of the biocontrol efficiency of Trichoderma asperellum against Fusarium wilt disease
Sterilized tomato seeds were sown in pots (8 cm) in diameter containing 28 g of sterilized peat moss and soil (1:2, w/w). Each pot contains five seeds, and the experiment included four treatments as follows:
1. Control (distilled water) only.
2. Pathogen (F. oxysporum) only.
3. Bioagent (T. asperellum) only.
4. Trichoderma + Fusarium.
Tomato plants were inoculated and kept at 25°C for 21 days, and the parameters of plant phenotype were measured, including the root length, shoot, and fresh weight. The shoots and the roots were dried in an oven at 70°C until constant weight (Mwangi et al., 2011).
Tomato root anatomy under biotic stress
The roots of tomato plants were sectioned and studied anatomically. The wax method was used in this experiment (Johansen, 1940). Briefly, plant roots were fixed for 72 h using FAA (formalin: acetic acid: alcohol 90:5:5) fixative. Then, roots were washed several times using distilled water and dehydrated using serial concentrations of alcohol 50, 70, 90, 95, and 100% (v/v), respectively. For clearing, they were transferred every 3 h from a mixture of 1:1 cedarwood oil: and absolute alcohol into pure cedarwood oil, followed by a mixture of cedarwood oil and xylene, and left overnight in pure xylene. Wax embedding was carried out in an oven adjusted at 60°C, embedded in clear wax, and sectioned using a rotatory microtome. Staining was done using safranine and fast green stains. Sections were mounted in a drop of Canada balsam, covered, and left to dry. The prepared slides of each root treatment were repeatedly examined under the light microscope (LEICA DM750) at the Microscopy Department, Faculty of Agriculture, Cairo University.
Trichoderma asperellum protects tomato fruits against FWD
Preparation of Trichoderma asperellum crude extract
Bioactive secondary metabolites of T. asperellum were extracted using ethyl acetate solvent, as previously described by Chen et al. (2018). Trichoderma was grown in a PDB medium and incubated on a rotary shaker (150 rpm) for 21 days at 28°C. After the incubation period, the fermented broth was filtered through Whatman filter paper No. 1, and the metabolites produced by the fungus were extracted from an equal volume of ethyl acetate. An equal volume of ethyl acetate was added to the filtrate and vigorously shaken for 5 min at room temperature. The mixtures were transferred to separating funnels, and the organic layers of ethyl acetate were allowed to separate from the aqueous layers. Then, the ethyl acetate layer was allowed to dry at room temperature, and the dried extracts were stored at 4°C for further use.
Assessment of extracted secondary metabolites against mycotoxigenic Fusarium oxysporum in tomato fruits
The antifungal activity of the T. asperellum extract was evaluated against Fusarium on tomato fruits, which were obtained from the supermarket, were initially washed under running water, sterilized for 2 min in 2% (v/v) sodium hypochlorite, and then rinsed with sterile water. Watery fruits were placed in plastic trays (sterilized with 70% (v/v) ethanol and under UV) and dried for 2 h under a laminar flow cabinet. The fruits were wounded with a sterile needle, and 20 μl of the pathogen spore suspension at 104 spores/mL was inoculated onto the wound. Subsequently, the drop was dried for 1 h. Finally, tomato fruits were treated with 20 μl of Trichoderma crude extract in the same wound where the pathogen inoculum had been applied. The treated tomato fruits were dried under a laminar flow cabinet until the droplet was completely dry, and the closed trays were incubated at room temperature (Stracquadanio et al., 2021; Maliehe et al., 2022). The untreated positive control was inoculated with the spore suspension of the pathogen, and the negative control was inoculated with Trichoderma extract. Fruits were monitored daily, and results were evaluated on the 11th day of incubation. The diameter of the lesions was also observed.
Mycotoxin analysis
Two grams of ground tomato fruits were mixed with 8 mL (80 acetonitrile (ACN): 20 H2O) and shaken for 20 min. Then, the mixture was centrifuged at 3500 rpm for 10 min and filtered through a 0.45 μm nylon filter. After filtration, the extract was diluted (1:4) with water containing 5 mM ammonium acetate. Analysis of mycotoxins (FB1, FB2, DON, and ZEN) was performed using liquid chromatography-electrospray ionization–tandem mass spectrometry (LC-ESI-MS/MS) with an ExionLC AC system for separation and SCIEX Triple Quad 5,500+ MS/MS system equipped with electrospray ionization (ESI) for detection. The instrument data were collected and processed using the SCIEX OS 1.6.10.40973 software. The targeted analytes were separated with an Agilent Zorbax Eclipse Plus C18 Column (4.6 × 100 mm, 1.8 μm) (Waskiewicz et al., 2010). The mobile phases consisted of two eluents containing 10 mM ammonium formate, eluent A was 0.1% (v/v) formic acid in the water, and eluent B was 0.1% (v/v) formic acid in methanol (LC grade). The mobile phase gradient was programmed as follows: 10% B at 0 min, 10–30% B from 0.0–2.0 min, 30–100% B from 2.0–11.0 min, 100% B from 11.0–11.5 min, 100–10% B from 11.5–12.0 min, 10% B from 12.0 to 15.0 min. The flow rate was 0.6 mL/min, and the injection volume was 10 μl. For MS/MS analysis, positive ionization mode (+MRM) was applied with the following parameters: curtain gas: 20 psi; collision gas: 9 psi; nebulizer current: 3; source temperature: 600°C; ion source gas 1 (nebulizer gas): 60 psi.
GC–MS analysis
The chemical composition of T. asperellum crude extract was assessed to detect the active constituents exhibiting antifungal activity. GC–MS was performed using a Trace GC1310-ISQ mass spectrometer (Thermo Scientific, Austin, TX, United States) with a direct capillary column TG–5MS (30 m × 0.25 mm × 0.25 μm film thickness). The column oven temperature was initially held at 50°C and then increased by 5°C/min to 230°C for 2 min, then increased to the final temperature of 290°C by 30°C /min and held for 2 min. The injector and MS, transfer line temperatures, were kept at 250°C and 260°C; Helium was used as a carrier gas at a 1 mL/min constant flow rate. The solvent delay was 3 min, and diluted samples of 1 μl were injected automatically using Autosampler AS1300 coupled with GC in the split mode. In full scan mode, EI/MS were collected at 70 eV ionization voltages over m/z 40–1,000. The ion source temperature was set at 200°C, and the components were identified by comparing their retention times and mass spectra with those of the WILEY 09 and NIST 11 mass spectral databases (Mulatu et al., 2022).
Statistical analysis
Analysis of variance (ANOVA) was assessed using IBM SPSS Statistics Version 28. The experiments were conducted using a completely randomized design with three replicates. The growth of the fungal strains was compared by t-test, and their biocontrol activities were evaluated by Fisher’s least significant difference (LSD) test at the 5% significance level.
Results
Isolation, morphological and molecular identification
Trichoderma asperellum was isolated from garlic rhizosphere soil by plate dilution, and the pure culture was maintained on a Potato dextrose agar (PDA) medium. Macroscopic morphology of T. asperellum revealed the rapid growth of the colony (3–4) days with 1–2 concentric rings. The mycelium, initially of a white color, acquired green and yellow shades or remained white due to the abundant production of conidia (Figure 1A). Regarding microscopic observations, T. asperellum showed globous, subglobous, or ovoid conidia, ampuliform phialides, and branched conidiophores, as shown in Figures 1B,C. The identification of the fungal isolate was further confirmed by analysis of the ITS sequence. One band of 531 bp was amplified using ITS primers, and the sequence alignments were performed using the Basic Local Alignment Search Tool (BLAST) to determine the phylogenetic positions of our strain with other Trichoderma strains on the GenBank. The fungal strain had (100%) matched identify with T. asperellum (Figure 1D). Moreover, the ITS sequence of T. asperellum was submitted to GenBank under the accession number (OQ130157).
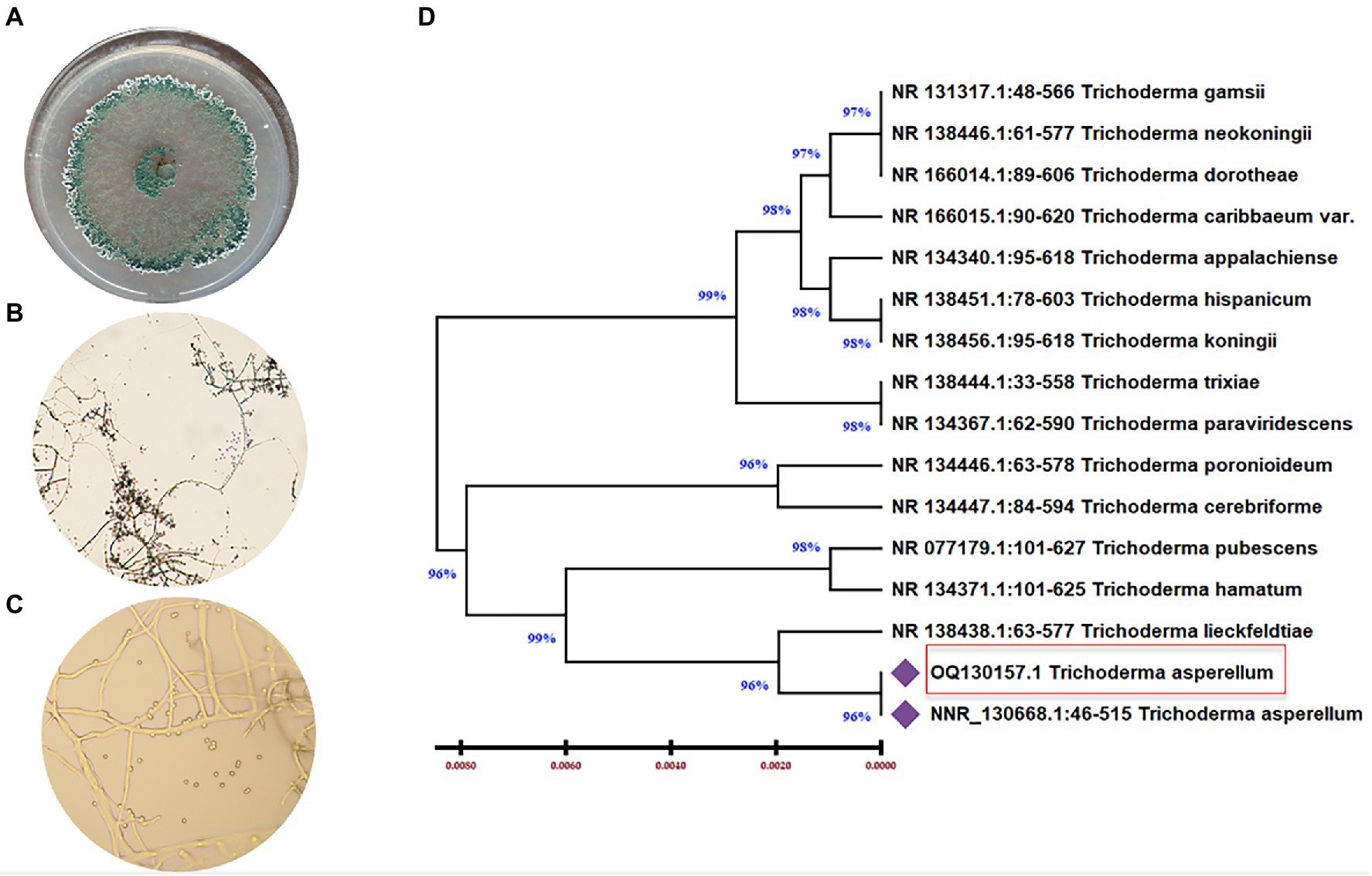
Figure 1. Morphological and molecular identification of T. asperellum. (A) The cultural view of T. asperellum grown on synthetic nutrient agar (SNA) media at 28°C for five days, which shows a ring around the original inoculum, (B,C) Microscopic view of conidia and conidiophores. (D) Molecular phylogenetic based on rDNA internal transcribed spacers (ITS) analyzes of T. asperellum by maximum Likelihood Model of MEGA11.0. The evolutionary distances were computed using the Maximum Composite Likelihood method and are in the units of the number of base substitutions per site. The proportion of sites where at least 1 unambiguous base is present in at least 1 sequence for each descendent clade is shown next to each internal node in the tree. This analysis involved 16 nucleotide sequences. All ambiguous positions were removed for each sequence pair (pairwise deletion option). The percentage of replicate trees in which the associated taxa clustered together in the bootstrap test (500 replicates) are shown next to the branches. The tree is drawn to scale, with branch lengths in the same units as those of the evolutionary distances used to infer the phylogenetic tree.
Evaluation of the antagonistic activity of Trichoderma asperellum against phytopathogens
Trichoderma asperellum was tested against several plant pathogenic fungi (F. oxysporum, F. solani, F. graminearum, A. alternata, and R. solani) to assess the antifungal activity using dual culture assay. Results showed that T. asperellum could inhibit the radial mycelial growth of all tested pathogens. The maximum growth rate inhibition (53.23%) was recorded against F. oxysporum followed by F. graminearum (45%), F. solani (43.19%), and R. solani (30.89%). In contrast, the lowest inhibitory activity percentage was exhibited against A. alternata (20.67%) (Table 1; Figure 2A). Based on the dual culture assay results, the highest growth rate inhibition was displayed against F. oxysporum. Therefore, different concentrations of sterilized culture filtrate of T. asperellum were tested against F. oxysporum to evaluate the optimum inhibiting concentration. Results showed that the inhibitory activity increased by increasing the sterilized culture filtrate concentration, and the maximum inhibitory activity percentage (59.38%) was observed at a concentration of 30% (Table 2; Figure 2B).
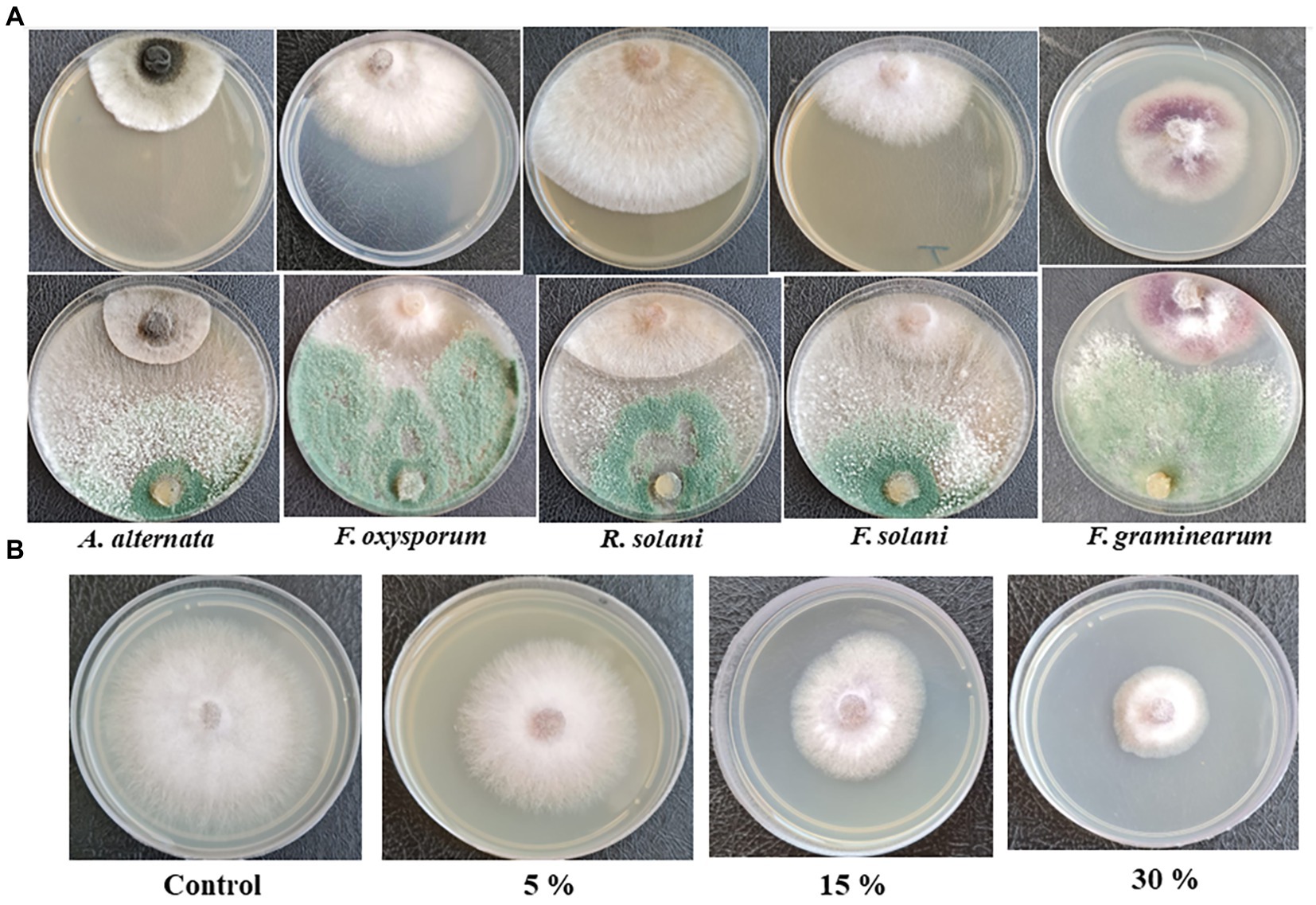
Figure 2. (A) Antagonistic activity of T. asperellum against plant pathogenic fungi in dual culture assay, (B) Effect of different concentrations of T. asperellum filtrate on F. oxysporum growth.
Scanning electron microscopy
The mycoparasitic nature of T. asperellum on F. oxysporum as a dual culture was examined by SEM (Figure 3). Interestingly, T. asperellum hyphae grew over the hyphae of F. oxysporum, followed by quick and excessive coiling. Finally, F. oxysporum lysis was eventually observed (Figures 3D–F).
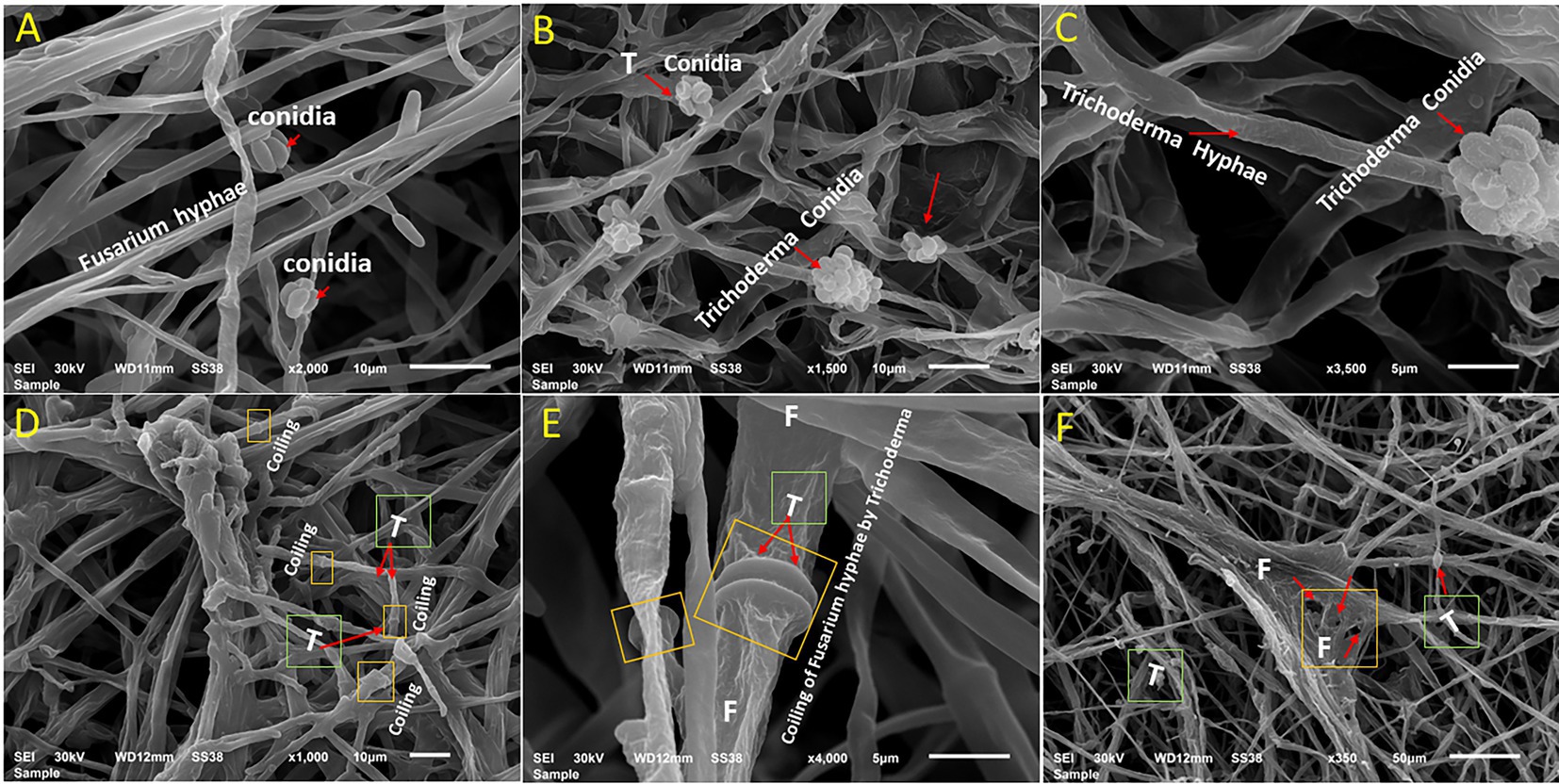
Figure 3. Scanning electron microscopy interpretations of the mycoparasitic of T. asperellum on F. oxysporum. (A) Fusarium pathogen alone, (B,C) T. asperellum alone (endophytic hyphae and conidia), (D) Mycelium of both fungi in contact via growing of Trichoderma hyphae (T) over Fusarium hyphae, (E) Coiling of Fusarium hyphae (F) by Trichoderma (T) as one of the antagonistic mechanisms (arrows), (F) Deformation of F. oxysporum by T. asperellum.
Plant growth-promoting and antifungal properties of Trichoderma asperellum
To assess the growth-promoting effects of T. asperellum on plant development, the plant growth hormone IAA was measured using a colorimetric assay. Results showed that the amount of IAA produced by T. asperellum was (12.5 ± 0.5742μgmL−1). Moreover, T. asperellum could solubilize phosphate, exhibiting phosphorus solubilization indices of 100% (Figures 4E,F). Additionally, T. asperellum showed high chitinase activity in a colloidal chitin medium supplemented with bromocresol purple (with final acidic pH of 4.7). Trichoderma, breakdown chitin to N- acetyl glucosamine shifting pH toward the alkalinity, which changes the medium color to purple (Figures 4B,C).
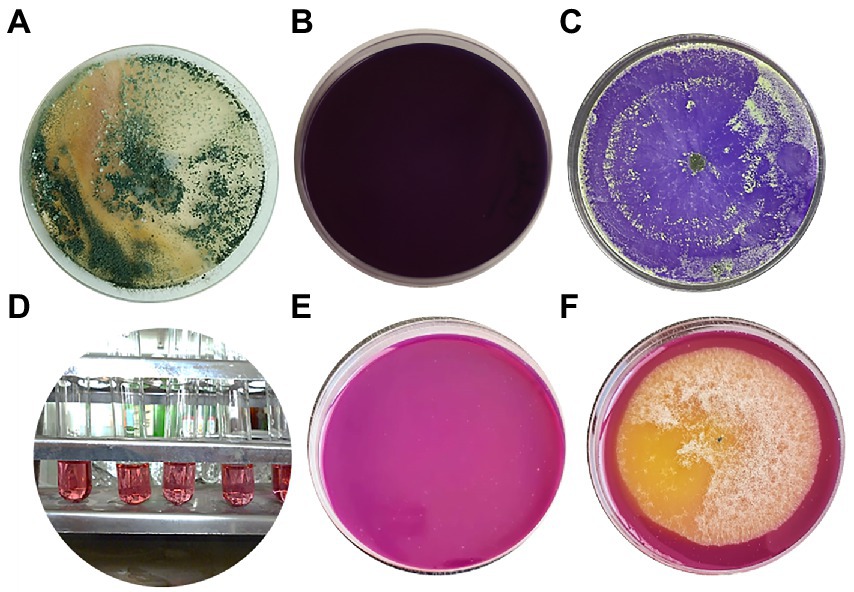
Figure 4. (A) Trichoderma asperellum strain grown on PDA at 28°C for seven days. (B,C) Screening of T. asperellum for chitinase activity on medium supplemented with colloidal chitin and control without inoculum. (D) IAA production by T. asperellum (5 replicates). (E,F) Phosphate solubilization of T. asperellum on NBRIP media supplemented with bromophenol blue as an indicator. (E) Uninoculated (control), (F) inoculated with T. asperellum.
Effect of Trichoderma asperellum on tomato seeds germination and growth promotion
Data illustrated in Figure 5; Supplementary Figure S1 indicated that T. asperellum treatment enhanced seed germination, root and shoot length, and vigor index in tomato seedlings. After 7 days, seeds treated with spore suspension of T. asperellum (106 spore mL−1) resulted in the highest seed germination rates (100%), as compared to the control (81.25%). Also, it can be seen that in the presence of T. asperellum grown on PDA in split interaction, the germination rate of seeds was enhanced (100%) as compared to an un-inoculated plate (66.6%) as presented in Figures 5C,D. In addition, T. asperellum inoculation promoted tomato growth parameters (Figures 5E,F). We observed that the Average shoot length of plants inoculated with T. asperellum simultaneously was 14.5% longer than the control. The average root length was also 17.6% longer than the control treatment (Figures 5G,H).
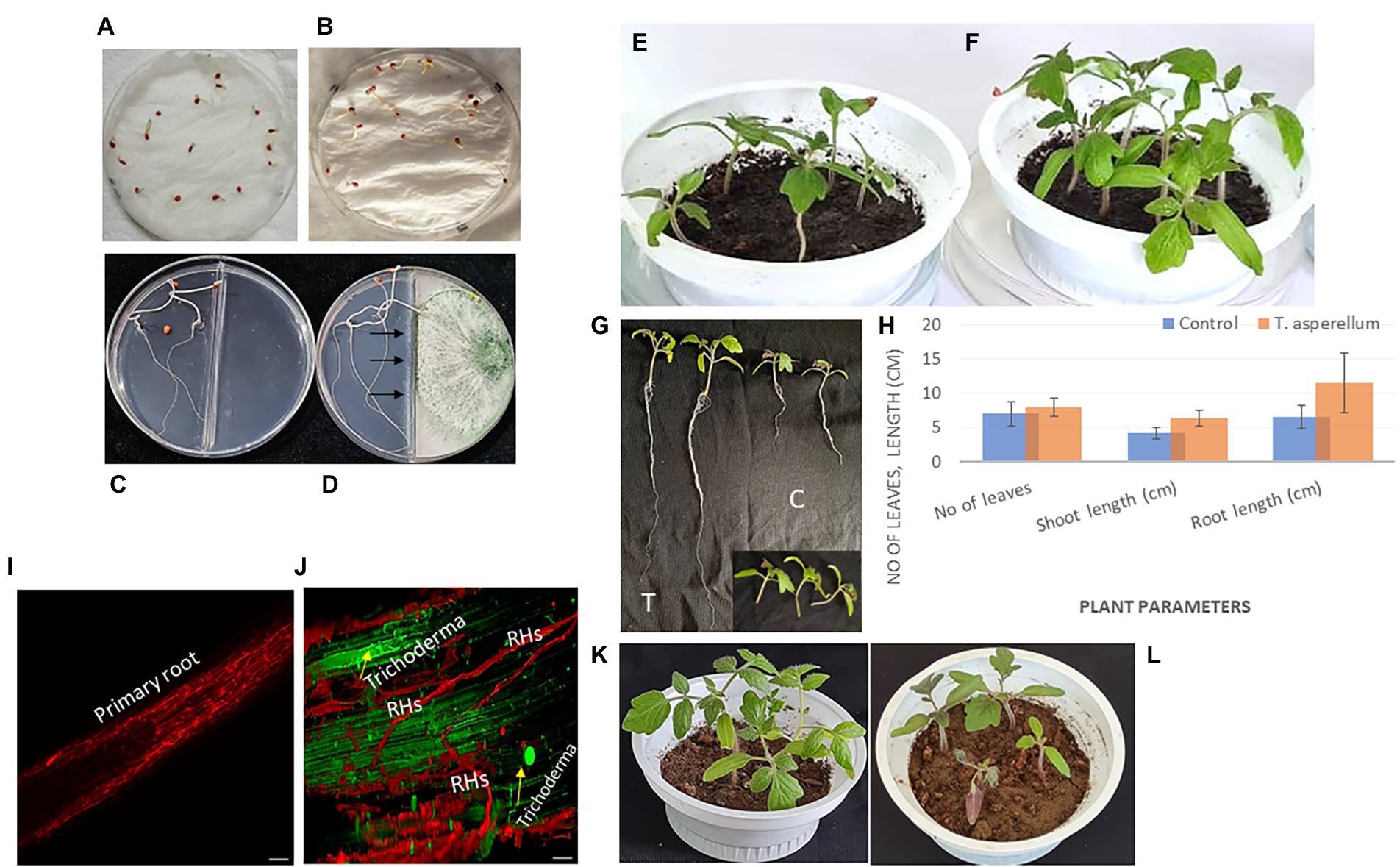
Figure 5. Trichoderma asperellum colonizes a tomato plant’s root system and promotes root elongation. (A) Seeds treated with distilled water. (B) Seeds treated with a spore suspension of T. asperellum. (C) Split interaction of seeds growing on water agar medium without Trichoderma treatment (control). (D) Split interaction with Trichoderma treatment. (E,F) Tomato plants are grown in the presence of Trichoderma after seed coating (pot at right) and without microbial inoculation (control only) (pot at left). (G) Promotion of root and shoot length via Trichoderma inoculation (T) compared to untreated plants (C). (H) Plant parameters include the number of leaves, shoot length, and root length with Trichoderma inoculation and control treatment. In vivo tests indicated the growth-promoting potential of this fungal endophyte. Trichoderma could boost plant growth by activating either an individual or numerous mechanisms. (I,J) Representative confocal laser scanning microscopical analysis illustrating the early and robust colonization of tomato roots/root hairs (RHs) by T. asperellum compared with the control (without microbial inoculation) using CLSM with a Leica TCS SP2. (K,L) Tomato plants are grown in the presence of T. asperellum after three weeks from seed coating (pot at left) and plants infected with Fusarium (pot at right).
Confocal microscopy
The capacity of the fungus T. asperellum to colonize tomato roots and stimulate plant growth was observed using a confocal imaging assay. Sterilized tomato seeds were inoculated with Trichoderma spores before germination on Petri dishes (150 mm) and kept in the dark for 3 days to study the early stages of the fungal root colonization. Interestingly, the root elongation of tomato seedlings and intercellular hyphal growth were observed compared with the uninoculated treatment (control) (Figures 5I,J). To obtain more insight, an in vitro experiment was carried out to study the effect of a biocontrol agent at the initial stage of root colonization by T. asperellum to understand its influence on tomato growth promotion. After inoculation, tomato roots were covered with conidia. Initial contact between conidia and the root was primarily at the region of root hairs.
Effect of Trichoderma asperellum on the growth of tomato plants under biotic stress of Fusarium oxysporum
There was a notable variation between the tomato plants inoculated with Trichoderma and Fusarium individually, as shown in Figure 6A. Data presented in Figures 5K,L showed that T. asperellum improved the growth of tomato plants under Fusarium infection. In addition, Figures 6B–D showed a significant increase in the root length and the fresh weights of shoots between the control (untreated) and the inoculated tomato plants with Trichoderma under biotic stress. The inhibitory effects of Trichoderma inoculation against Fusarium invasion were demonstrated in Figure 6A. Fusarium pathogen invaded the root system and significantly decreased roots and shoot dry weights, the number of leaves, and root length. In addition, there was a marked increase in the root length and shoot fresh weight during the Trichoderma-Fusarium interactions (Figures 6C–D). T. asperellum controlled the FWD caused by F. oxysporum by enhancing the plant phenotypes, including shoot and root length (cm), shoot and fresh root weight (g), shoot and root dry weight (g), as well as the number of leaves. It can be seen that number of leaves in plants inoculated with Trichoderma was (45%) higher than the number of leaves in the treated plants with Fusarium alone.
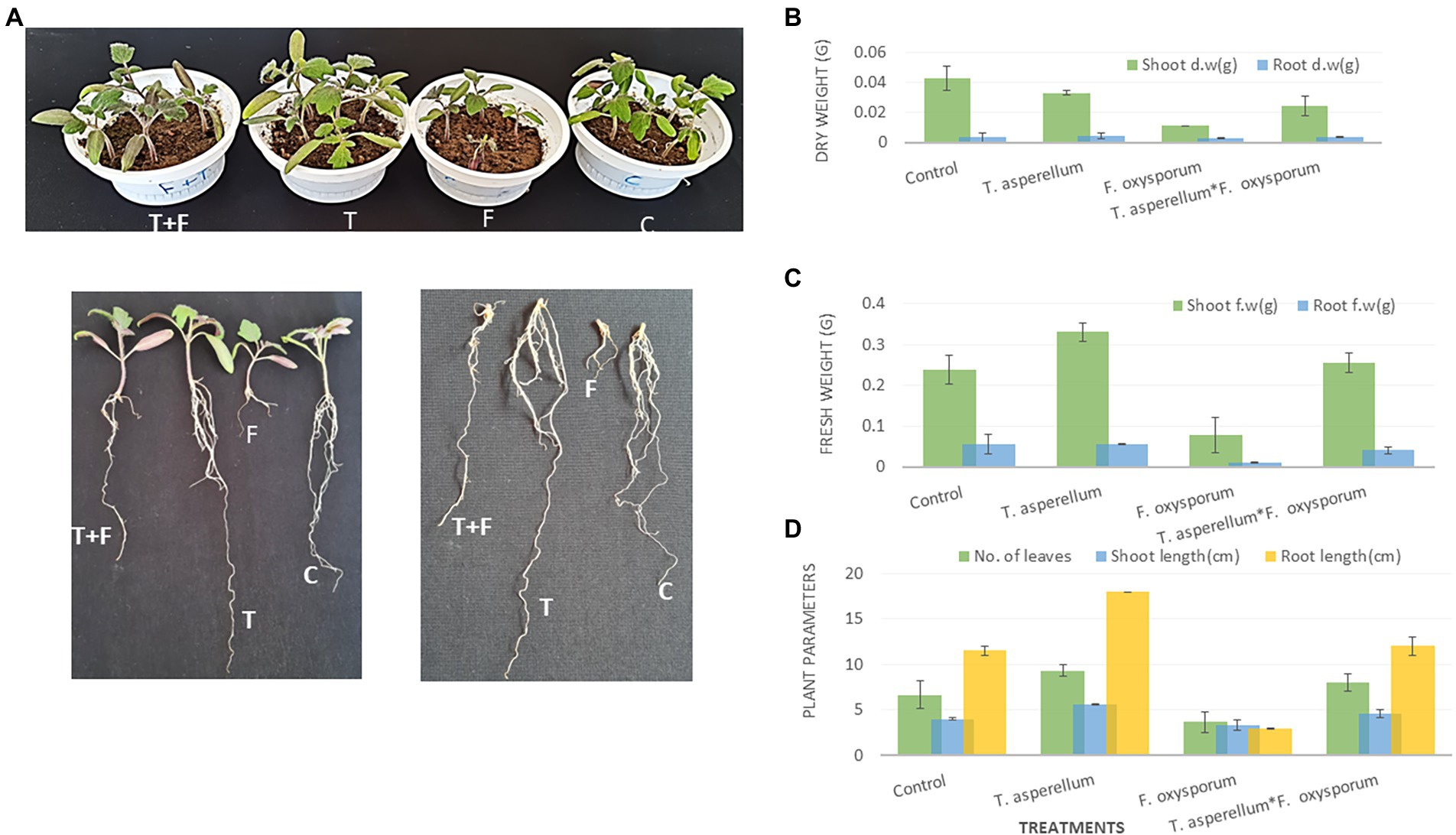
Figure 6. The Effect of T. asperellum on the growth of tomato plants under biotic stress of F. oxysporum including (A); T-Trichoderma enhances both root and shoot of tomato seedlings, F-Fusarium can penetrate tomato roots and cause wilting or root rotting diseases, T + F-the interaction between the fungal endophyte and the pathogen, C-tomato plants without microbial treatment. Shoot and root dry weight (B). Shoot and root fresh weight and (C). The number of leaves, shoot, and root length (cm) (D).
Root anatomy
Transverse sections of tomato roots treated with T. asperellum and the interactions between bioagent and the fungal pathogen were studied to evaluate their impact on root health. Interestingly, the inoculation of tomato roots with Trichoderma increased whole root thickness and the number of xylem vessels compared to the control (Figures 7A,D,G). The highest degradation area of the root cortex was observed in plants infested with F. oxysporum. Lateral root (LR) development was also detected in the treated tomato roots with T. asperellum (Figure 7F). However, the combination of T. asperellum and F. oxysporum enhanced the root thickness in the cortex of the tomato tap root (Figure 7I). Light microscope sections showed that Trichoderma enhanced the cortex and xylem diameters. It was also noted that the xylem and the endodermis diameters were thinner in control roots than in other treatments; this trend was the same in both hybrids (Figures 7C,F,I).
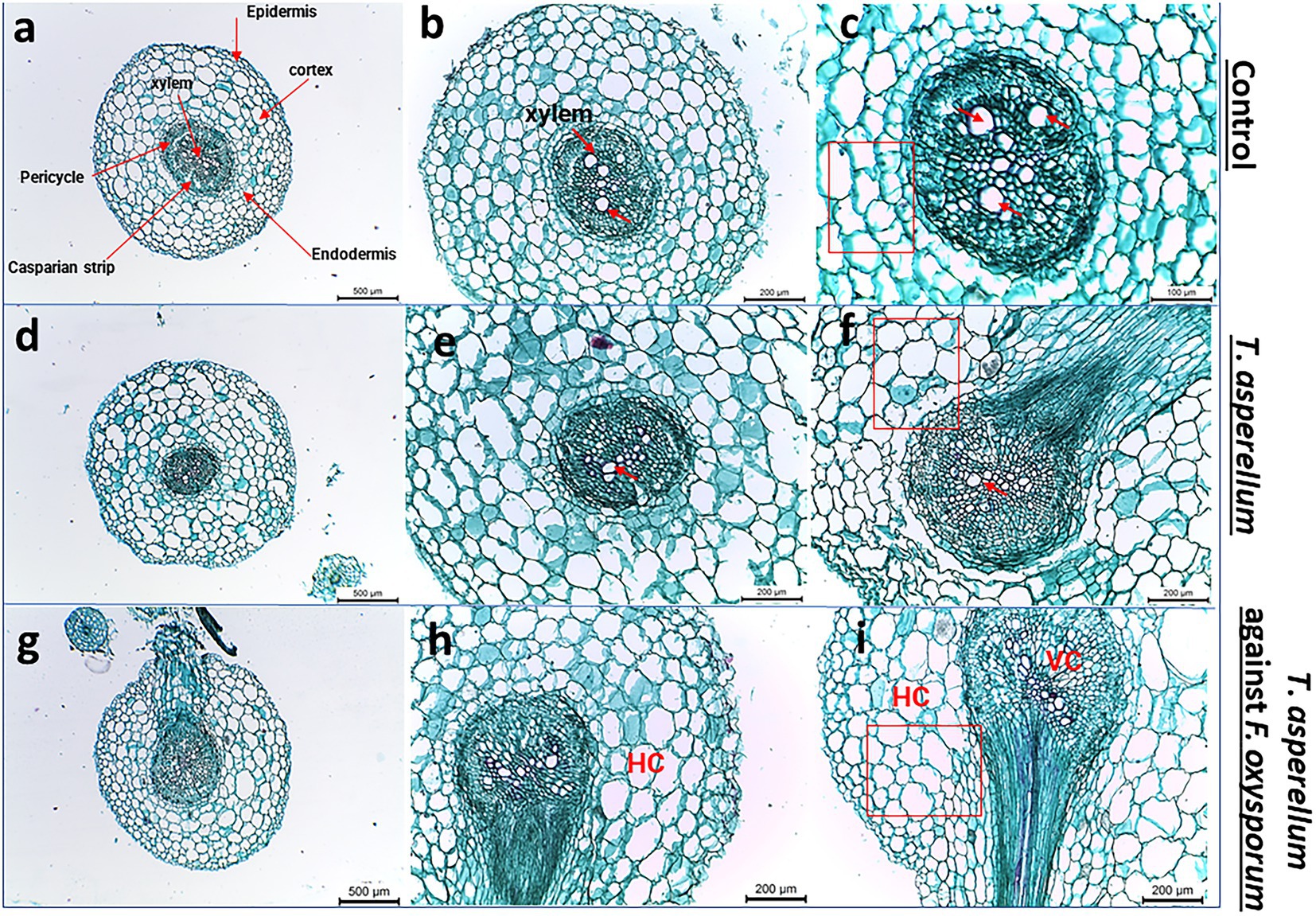
Figure 7. Light microscope images of tomato root cross sections near the root tip taken from plants grown under biotic stress. (A–C) Healthy tomato root, (D–F) Transverse sections of tomato roots plant treated with Trichoderma, (G–I) shows the root cross-sections after applying Trichoderma against Fusarium pathogen. Red arrows indicate the xylem vessels.
Biocontrol efficiency on tomato fruits against FWD
The disease control efficacy of T. asperellum against F. oxysporum is shown in Figures 8A–C. After 11 days of storage, tomato fruits inoculated with F. oxysporum and treated with T. asperellum extract showed an increase in shelf-life compared with the untreated control (Fusarium only). Moreover, tomato fruits inoculated with Trichoderma metabolites showed a significant reduction in lesion diameter compared to the pathogen-treated fruits (Figure 8). Results indicated that T. asperellum exhibited a significant inhibitory effect against F. oxysporum. In addition, the mycotoxins typically produced by Fusarium species (FB1, FB2, ZEN, and DON) were not detected by LC–MS/MS analysis, even in the untreated controls.
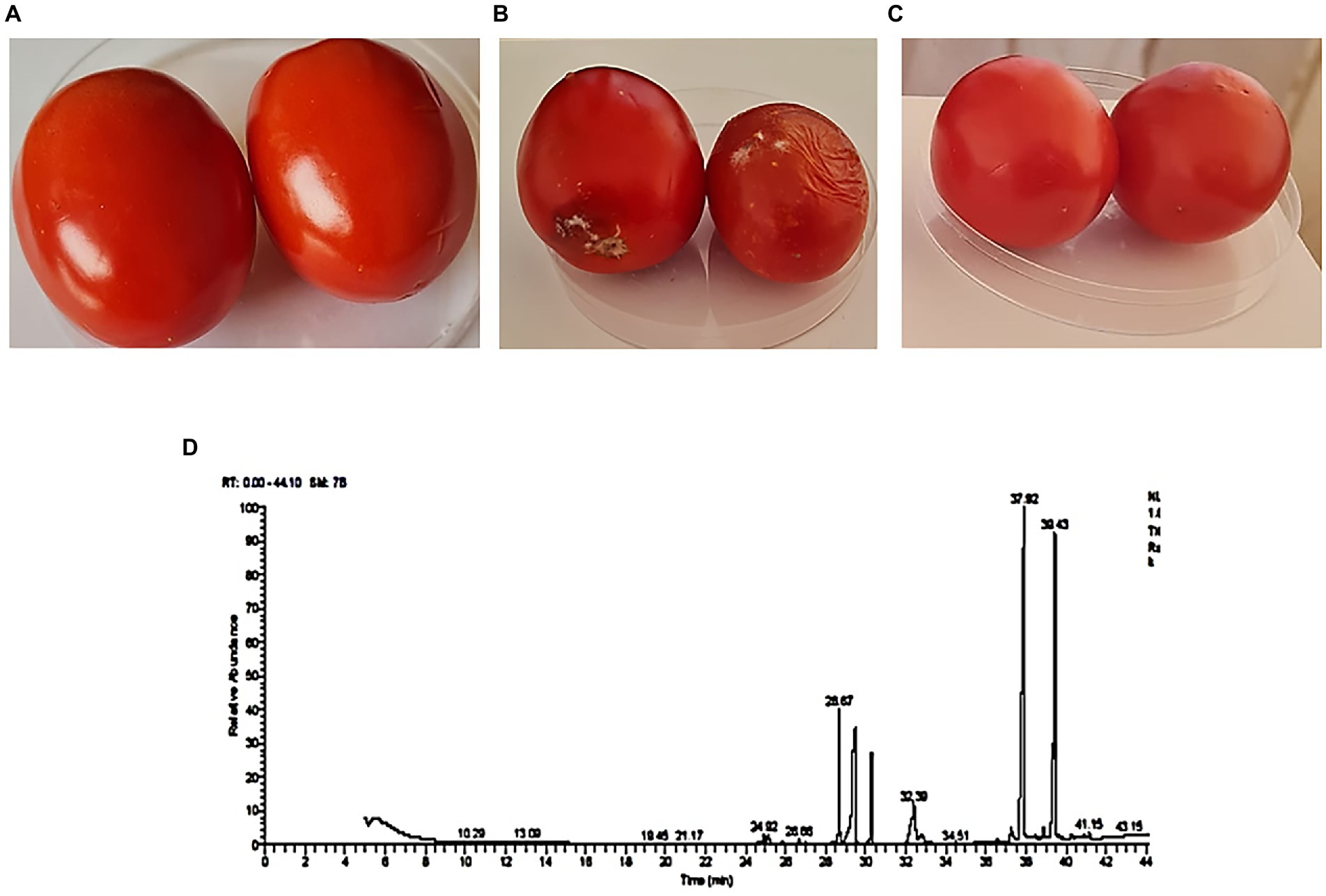
Figure 8. Biocontrol efficacy of T. asperellum crude extract against F. oxysprum. (A) Tomato fruit inoculated with T. asperellum extract. (B) Tomato fruit inoculated with a spore suspension of F. oxysporum only. (C) Tomato fruit inoculated with T. asperellum extract and F. oxysporum. (D) The fraction of bioactive secondary metabolites were detected using a GC–MS chromatogram obtained from T. asperellum.
GC–MS analysis
The GC–MS analysis of the ethyl acetate extract of T. asperellum revealed the presence of different bioactive compounds (Figure 8D). The compound name, molecular formula (MF), molecular weight (MW), concentration (peak area %), and retention time (RT) are present in Table 3. Interestingly, the most predominant compounds were 1,2-Benzenedicarboxylic acids (32.15%), Palmitic Acid, TMS derivative (17.05%), D-Glucopyranose, 5TMS derivative(7.04%), Myo-Inositol, 6TMS derivative(4.55%), Oleic Acid, (Z)-, TMS derivative (2.82%), 9,12,15-OCTADECATRIENOIC ACID, 2-[(TRIMETHYLSILYL) OXY]-1-[[(TRIMETHYLSILYL) OXY] MET HYL]ETHYL ESTER, (Z, Z, Z)-(1.35%), 4H-1-Benzopyran-4-one,2-(3,4-Dihydroxyohenyl)-6,8-DI-á-D-Glucopyranosyl-5, 7-Dihydroxy (1.35%) and Stearic acid, TMS derivative (0.82%).
Discussion
Various antagonistic activities were studied against different fungal pathogens using T. asperellum, as shown in Figure 3. This was sufficient evidence to conclude that the Trichoderma strain could be a bio-control agent and stimulate plant health via secondary metabolites and hormone production. In addition, this strain has the capability to sustain beneficial interaction with the host plant under stressful biotic conditions. Several Trichoderma isolates have been reported to suppress FWD (Srivastava et al., 2010; Marzano et al., 2013). Trichoderma species also have the potential of antagonistic interactions with different plant pathogens, which determines the biocontrol efficiency of the fungal pathogens. Mycoparasitism, antibiosis, competition for nutrients, and induced systemic resistance in plants are diverse antifungal activities of Trichoderma spp. (Druzhinina et al., 2011; Modrzewska et al., 2022). Therefore, these studies showed the successful application of Trichoderma in the field, which could impair plant health and productivity and suppress the Fusarium wilt disease. Additionally, this study investigated the contribution of T. asperellum to P solubilization and IAA production. Experiments were conducted in duplicate to investigate the ability of Trichoderma to promote plant growth. Trichoderma produced a clear halo around its colonies, which solubilized soluble P in vitro. Interestingly, T. asperellum released an auxin-like phytohormone that significantly increased the total root length of tomato plants. Since the tomato is one of the most produced crop plants growing in fields and greenhouses worldwide, this study evaluated the mechanism by which Trichoderma assisted tomato roots in response to Fusarium infection during plant growth. Plant roots exploit morphological plasticity to adapt and respond to different soil environments (Alaguero-Cordovilla et al., 2018). However, most of the investigated Trichoderma species colonize the root surface or inhabit inside root tissues as endophytes (Bailey et al., 2006). Trichoderma application with tomato seedlings shows adequate protection against F. oxysporum. Interestingly, the T. asperellum also benefitted plant growth promotion via root elongation during the pathogen attacks (Figure 5G). This is consistent with the dual cultural assay data between the biological agents and the pathogen infection, which decreased the hyphal growth. The results showed that the highest growth rate inhibition was displayed against F. oxysporum, causing FWD.
A precise in vitro experimental design was established to explore the impact of Trichoderma inoculation on the tomato root system during early growth (Figure 5D). Our results revealed that the biocontrol agent led to observed differences during early growth. The treatments were categorized into tomato roots with no microbial inoculation (control) and root inoculation with Trichoderma. The effect of Trichoderma strain on plant growth under abiotic stress was initially evaluated by measuring the root and shoot length and the number of leaves. In addition, the fungal inoculation significantly increased the plant parameters compared to untreated plants (control), as shown in Figure 5H. In that context, some studies have revealed that the inoculation of T. harzianum SQR-T037 improved the growth of tomato plants under greenhouse and field conditions (Cai et al., 2013, 2015).
The root system is essential for plant growth because of its basic functions in the selective absorption of water and nutrients (Karthika et al., 2018; Das et al., 2022), storage organ, and a selective barrier against pathogens (Wiedemeier et al., 2002; Atkinson et al., 2014). Characterization of root morphology and cellular development of two tomato species, S. pennellii and S. lycopersicum ‘M82’ during early growth were studied by Ron et al. (2013). This study provided significant differences in an extensive range of root traits with developmental significance. In addition, the newly emerged lateral roots (LRs) were significantly correlated with Trichoderma inoculation in tomato plants (Figure 7F). In addition, Trichoderma (green) colonized tomato roots (red), which visualized its mobility by the confocal imaging microscope (Figure 5J). It is well known that Trichoderma strains are able to colonize plant roots and stimulate the growth via the production of phytohormones, thus playing a crucial role in regulating the plant root system (Benítez et al., 2004; Harman et al., 2004; Cai et al., 2013). These findings agreed with our observations which suggested that improving plant growth occurs through a direct effect of Trichoderma species on root development.
Furthermore, Trichoderma can regulate plant growth through other mechanisms, such as mineral solubilization, to sustain plant health. These findings were consistent with the results found in this study when inoculating tomato seeds with T. asperellum in healthy conditions or under pathogenic stress. Tomato root colonization and Trichoderma mobility were visualized using confocal laser imaging (Figure 5J) in the rhizosphere to discover the tomato root-Trichoderma interactions. Tomato seeds were sterilized, germinated, and inoculated, and seedlings were grown under the conditions described (Simons et al., 1996). Trichoderma and Fusarium were labeled with autofluorescent protein (AFP) markers to visualize their interactions using confocal laser scanning microscopy (CLSM). This study better understood the biocontrol interaction and mycoparasitic activity (Bloemberg, 2007).
Similarly, our study showed the fungal mobility in the tomato rhizosphere, which stimulates root growth promotion (Bolwerk and Lugtenberg, 2005), as shown in Figures 5I,J, which illustrates the early and robust stage of tomato root colonization. In addition to direct parasitism of plant pathogens, interactions with Trichoderma enhance plant fitness in response to biotic and abiotic stresses (Bolwerk et al., 2005; Hermosa et al., 2012). All microbes generally hunt for food, of which the root supplies a substantial amount of exudate. Visualization of plant-microbe and microbe-microbe interactions during the plant developmental stages is essential to understanding the underlying mechanisms behind the root exudates/signaling and microbial colonization via tracking microbial mobility. In addition, it explores the various mechanisms of biological control by increasing tolerance to abiotic stress and stimulating defense strategies against fungal pathogens (Hermosa et al., 2012). Tomato seedlings inoculated with Burkholderia tropica strain MTo-293 exhibited some activities in plant growth promotion and biological control under greenhouse conditions (Bernabeu et al., 2015). They studied the performance of the microbial colonization of different vegetal tissues. A later confocal microscopy study also revealed that T. harzianum could colonize the epidermis and cortex of tomato roots (Chacón et al., 2007). In a recent study, T. harzianum T-78 enhanced the resistance of tomato plants against the root-knot nematode Meloidogyne incognita through priming for salicylic acid (SA)-and jasmonic acid (JA)-regulated defenses (Martínez-Medina et al., 2017). Root colonization by Trichoderma requires a complex molecular dialog between the fungus and plant. This colonization is limited to the outermost layers of the root and does not penetrate the plant vascular bundle, which increases the resilience of abiotic stresses (i.e., salinity and drought). In addition, improving the capacity to absorb nutrients and actively stimulates plant growth (Contreras-Cornejo et al., 2021). T. atroviride AN35 and T. cremeum AN392 colonized the roots of wheat plants. The microscopic imaging showed that the hyphae of the Trichoderma grew on the root surface of wheat and corn (Zea mays) seedlings (Contreras-Cornejo et al., 2018). This behavior was also observed in T. atroviride during the colonization of Arabidopsis roots (Salas-Marina et al., 2011). A study by Ruano-Rosa et al. (2016) showed the root colonization process between T. harzianum and olive crops.
Trichoderma species are known to produce a wide range of bioactive secondary metabolites that are known to have antifungal, antibacterial, and toxic properties to control a wide range of phytopathogens, such as Fusarium species, Botrytis cinerea, Pythium species, Rhizoctonia solani, Sclerotinia sclerotiorum, and Ustilago maydis (Hermosa et al., 2014; Khan et al., 2020). Analysis by GC –MS is essential for the identification of natural compounds of the microbe to explore the underlying mechanisms of the antifungal activity (Siddiquee et al., 2012; Qualhato et al., 2013; Meena et al., 2017). Usually, volatile compounds are identified, such as aromatic compounds, fatty acids, general hydrocarbons, and hydroxy or amino compound metabolites (Siddiquee et al., 2012). In the present study, 18 bioactive compounds were detected in the crude extract of T. asperellum by GC–MS analysis. The most commonly identified compounds were fatty acids and their derivatives, esters such as 1,2-Benzenedicarboxylic acid, Palmitic acids, Oleic acid, 9-Octadecenoic acid (E), methyl ester, and 9,12,15-Octadecatrienoic acid, methyl ester, (Z, Z, Z), sugars (D-Glucopyranose) and sugar alcohol (Myo-Inositol). It was investigated that sugars are essential to fuel the energy required for defenses and serve as signals for regulating defense genes in plant-microbe interactions (Roitsch et al., 2003; Bolton, 2009). Myo-inositol plays essential roles in stress responses, development, and many other processes (Michell, 2008; Valluru and Van den Ende, 2011). Moreover, the volatile metabolites play essential roles in mycoparasitic interactions between Trichoderma and plants (Altieri et al., 2009) 1,2 Benzenedicarboxylic acid has antifungal activity (Chen et al., 2020). Liu et al. (2008) emphasized the antifungal activity of palmitic acid against four economically important phytopathogenic fungi (Alternaria solani, Colletotrichum lagenarium, Fusarium oxysporum f. sp. cucumerinum, and F. oxysporum f. sp. lycopersici). Walters et al. (2004) examined the antifungal ability of oleic acid against plant pathogenic fungi. They verified that a 1,000 μM could reduce the mycelial growth of all tested fungi (Rhizoctonia solani, Pythium ultimum, Pyrenophora avenae, and C. perniciosa). The primary mechanism of the antifungal action of fatty acids states that fatty acids insert themselves into the lipid bilayers of fungal membranes compromising membrane integrity, resulting in an uncontrolled release of intracellular electrolytes and proteins, eventually leading to cytoplasmatic disintegration of fungal cells (Avis and Bélanger, 2001). Thus, these fatty acids play a vital role in the extract of T. asperellum by controlling the growth of F. oxysporum. Therefore, it protects tomato plants from Fusarium diseases.
Surprisingly, the mycotoxins naturally produced by Fusarium species (FB1, FB2, ZEN, and DON) were not detected by LC–MS analysis, even in the untreated controls. Our results agree with Stracquadanio et al. (2021), who showed that F. graminearum had no mycotoxin production in tomato fruits. Likewise, in the studies by Lori et al. (2003) and Haidukowski et al. (2005) on the same strain, F. graminearum ITEM 126 showed mycotoxin production in contaminated wheat kernels. This could be because this strain does not produce mycotoxins when infecting tomatoes but only in small grain cereals and maize (Munkvold, 2017).
Conclusion
Tomato is a global economically essential vegetable crop. However, FOL is the causal agent of the FWD of tomato crops. Biological control offers a promising eco-friendly method to manage this disease. The Trichoderma strain is successfully used as a bio-control agent because it stimulates the plant immune system against pathogen attacks. Its growth-promoting ability in soil provides an additional benefit in the agricultural application of fertilizers and antifungal activity. Furthermore, a combination of the in vitro and planta experiments will improve the role of this fungus on plant performance. In addition, the fungus successfully promotes plant growth in controlled conditions. The plant growth-promoting traits and biocontrol efficiency of the Trichoderma strain were also evaluated based on the recurring role of T. asperllum as a biocontrol agent against various fungal pathogens. T. asperellum displayed the highest antagonistic activity against F. oxysprum at 53.24%, and 30% free cell filtrate inhibited F. oxysporum at 59.39% as well. This study provides vital insights into the plant-microbe interaction and the microbe-microbe interactions, including diverse antagonistic mechanisms. For instance, chitinase activity, IAA, and P solubility were observed in vitro assays, indicating Trichoderma’s capability to control the fungal pathogen and enhance plant growth. Additionally, using confocal microscopy, tomato root colonization was visualized to understand the mobility of the Trichoderma strain in the host plant. These data suggest microbial application in seed coating or foliar spraying, which may improve food safety by applying beneficial microbes. In sum, improving the efficacy and development of biocontrol agents to help small farmers will increase their crop productivity in an accessible and economical way. Thereby positively impacting farmers’ profits and sustaining the food safety approaches. In addition, developing sustainable crops that could be grown with little to no pesticides and/or chemical fertilizers reduces costs for farmers in developing areas. Moreover, developing novel and environmentally friendly approaches is essential to reduce the FWD incidence and yield loss in tomato crops. Therefore, more field applications with this Trichoderma strain are required. In addition, the transcriptome profiles of the root system following microbial treatment and the influence of endophytic fungi on tomato metabolism.
Data availability statement
The datasets presented in this study can be found in online repositories. The names of the repository/repositories and accession number(s) can be found in the article/Supplementary material.
Author contributions
AS, RA, KA, and OH designed the study. AS, RA, and OH wrote the original manuscript. AS and RA conducted all the in vitro experiments, confocal work, and collected the data. AS, RA, and OH conducted the in planta assays. OH performed the ITS sequencing to confirm fungal genus taxonomy. OH, KA, and MA performed the statistical analysis and edited the manuscript. All authors contributed to the article and approved the submitted version.
Conflict of interest
The authors declare that the research was conducted in the absence of any commercial or financial relationships that could be construed as a potential conflict of interest.
Publisher’s note
All claims expressed in this article are solely those of the authors and do not necessarily represent those of their affiliated organizations, or those of the publisher, the editors and the reviewers. Any product that may be evaluated in this article, or claim that may be made by its manufacturer, is not guaranteed or endorsed by the publisher.
Supplementary material
The Supplementary material for this article can be found online at: https://www.frontiersin.org/articles/10.3389/fmicb.2023.1140378/full#supplementary-material
Footnotes
References
Agrawal, T., and Kotasthane, A. S. (2012). Chitinolytic assay of indigenous Trichoderma isolates collected from different geographical locations of Chhattisgarh in Central India. Springerplus 1, 1–10. doi: 10.1186/2193-1801-1-73
Akbar, A., Hussain, S., and Ali, G. S. (2018). Germplasm evaluation of tomato for resistance to the emerging wilt pathogen Fusarium equiseti. J. Agric. Stud 5:174. doi: 10.5296/jas.v6i3.13433
Alaguero-Cordovilla, A., Gran-Gómez, F. J., Tormos-Moltó, S., and Pérez-Pérez, J. M. (2018). Morphological characterization of root system architecture in diverse tomato genotypes during early growth. Int. J. Mol. Sci. 19:3888. doi: 10.3390/ijms19123888
Almeida, F., Rodrigues, M. L., and Coelho, C. (2019). The still underestimated problem of fungal diseases worldwide. Front. Microbiol. 10:214. doi: 10.3389/fmicb.2019.00214
Aloisio, F. C., Junior, F. B., Lillian, C., Luciane, D. O. M., and De Oliveira, J. O. (2019). Efficiency of Trichoderma asperellum UFT 201 as plant growth promoter in soybean. Afr. J. Agric. Res. 14, 263–271. doi: 10.5897/AJAR2018.13556
Altieri, C., Bevilacqua, A., Cardillo, D., and Sinigaglia, M. (2009). Antifungal activity of fatty acids and their monoglycerides against Fusarium spp. in a laboratory medium. Int. J. Food Sci. Technol. 44, 242–245. doi: 10.1111/j.1365-2621.2007.01639.x
Atkinson, J. A., Rasmussen, A., Traini, R., Voß, U., Sturrock, C., Mooney, S. J., et al. (2014). Branching out in roots: uncovering form, function, and regulation. Plant Physiol. 166, 538–550. doi: 10.1104/pp.114.245423
Attia, M. S., Abdelaziz, A. M., Al-Askar, A. A., Arishi, A. A., Abdelhakim, A. M., and Hashem, A. H. (2022). Plant growth-promoting fungi as biocontrol tool against Fusarium wilt disease of tomato plant. J. Fungi 8:775. doi: 10.3390/jof8080775
Avis, T. J., and Bélanger, R. R. (2001). Specificity and mode of action of the antifungal fatty acid cis-9-heptadecenoic acid produced by Pseudozyma flocculosa. Appl. Environ. Microbiol. 67, 956–960. doi: 10.1128/AEM.67.2.956-960.2001
Awad, N. E., Kassem, H. A., Hamed, M. A., El-Feky, A. M., Elnaggar, M. A. A., Mahmoud, K., et al. (2018). Isolation and characterization of the bioactive metabolites from the soil derived fungus Trichoderma viride. Mycology 9, 70–80. doi: 10.1080/21501203.2017.1423126
Bailey, B. A., Hanhong Bae, M. D., Strem, D. P. R., Thomas, S. E., Crozier, J., Samuels, G. J., et al. (2006). Fungal and plant gene expression during the colonization of cacao seedlings by endophytic isolates of four Trichoderma species. Planta 224, 1449–1464. doi: 10.1007/s00425-006-0314-0
Barroso, F. M., Muniz, P. H. P. C., Milan, M. D., Silva, W., dos Santos, N., de Faria, C., et al. (2019). Growth promotion of parsley (Petroselinum crispum L.) using commercial strains of Trichoderma spp. J. Agric. Sci. 11, 493–499. doi: 10.5539/jas.v11n4p493
Bawa, I. (2016). Management strategies of Fusarium wilt disease of tomato incited by Fusarium oxysporum f. sp. lycopersici (Sacc.) a review. Int. J. Adv. Acad. Res 2, 1–16.
Benítez, T., Rincón, A. M., Carmen Limón, M., and Codon, A. C. (2004). Biocontrol mechanisms of Trichoderma strains. Int. Microbiol. 7, 249–260.
Bernabeu, P. R., Pistorio, M., Torres-Tejerizo, G., Estrada-De, P., Santos, L., Galar, M. L., et al. (2015). Colonization and plant growth-promotion of tomato by Burkholderia tropica. Sci. Hortic. 191, 113–120. doi: 10.1016/j.scienta.2015.05.014
Bloemberg, G. V. (2007). Microscopic analysis of plant-bacterium interactions using auto fluorescent proteins. Eur. J. Plant Pathol. 119, 301–309. doi: 10.1007/978-1-4020-6776-1_6
Boccia, F., Di Donato, P., Covino, D., and Poli, A. (2019). Food waste and bio-economy: a scenario for the Italian tomato market. J. Clean. Prod. 227, 424–433. doi: 10.1016/j.jclepro.2019.04.180
Bolton, M. D. (2009). Primary metabolism and plant defense—fuel for the fire. Mol. Plant-Microbe Interact. 22, 487–497. doi: 10.1094/MPMI-22-5-0487
Bolwerk, A., Lagopodi, A. L., Lugtenberg, B. J. J., and Bloemberg, G. V. (2005). Visualization of interactions between a pathogenic and a beneficial Fusarium strain during biocontrol of tomato foot and root rot. Mol. Plant-Microbe Interact. 18, 710–721. doi: 10.1094/MPMI-18-0710
Bolwerk, A., and Lugtenberg, B. J. J. (2005). “Visualization of interactions of microbial biocontrol agents and phytopathogenic fungus Fusarium oxysporum f. sp. radicis-lycopersici on tomato roots,” in PGPR: Biocontrol and Biofertilization. ed. Z. A. Siddiqui (Dordrecht: Springer), 217–231.
Borrero, C., Trillas, M. I., Delgado, A., and Avilés, M. (2012). Effect of ammonium/nitrate ratio in nutrient solution on control of Fusarium wilt of tomato by Trichoderma asperellum T34. Plant Pathol. 61, 132–139. doi: 10.1111/j.1365-3059.2011.02490.x
Buriro, M., Oad, F. C., Keerio, M. I., Tunio, S., Gandahi, A. W., Hassan, S. W. U., et al. (2011). Wheat seed germination under the influence of temperature regimes. Sarhad J. Agric. 27, 539–543.
Cai, F., Chen, W., Wei, Z., Pang, G., Li, R., Ran, W., et al. (2015). Colonization of Trichoderma harzianum strain SQR-T037 on tomato roots and its relationship to plant growth, nutrient availability and soil microflora. Plant Soil 388, 337–350. doi: 10.1007/s11104-014-2326-z
Cai, F., Guanghui, Y., Wang, P., Wei, Z., Lin, F., Shen, Q., et al. (2013). Harzianolide, a novel plant growth regulator and systemic resistance elicitor from Trichoderma harzianum. Plant Physiol. Biochem. 73, 106–113. doi: 10.1016/j.plaphy.2013.08.011
Chacón, M. R., Rodríguez-Galán, O., Benítez, T., Sousa, S., Rey, M., Llobell, A., et al. (2007). Microscopic and transcriptome analyses of early colonization of tomato roots by Trichoderma harzianum. Int. Microbiol. 10:19. doi: 10.2436/20.1501.01.4
Chen, X., Huang, Y., and Huang, Z. (2018). Combination of ethyl acetate extract of Trichoderma hamatum fermentation broth and fungicide carbendazim enhances inhibition against Scleromitrula shiraiana under laboratory conditions Pakistan. J. Zool. 50, 2239–2247. doi: 10.17582/journal.pjz/2018.50.6.2239.2247
Chen, J. H., Xiang, W., Cao, K. X., Lu, X., Yao, S. C., Hung, D., et al. (2020). Characterization of volatile organic compounds emitted from endophytic Burkholderia cenocepacia ETR-B22 by SPME-GC-MS and their inhibitory activity against various plant fungal pathogens. Molecules 25:3765. doi: 10.3390/molecules25173765
Contreras-Cornejo, H. A., Macías-Rodríguez, L., Del-Val, E., and Larsen, J. (2016). Ecological functions of Trichoderma spp. and their secondary metabolites in the rhizosphere: interactions with plants. FEMS Microbiol. Ecol. 92:fiw036. doi: 10.1093/femsec/fiw036
Contreras-Cornejo, H. A., Macías-Rodríguez, L., Del-Val, E., and Larsen, J. (2018). The root endophytic fungus Trichoderma atroviride induces foliar herbivory resistance in maize plants. Appl. Soil Ecol. 124, 45–53. doi: 10.1016/j.apsoil.2017.10.004
Contreras-Cornejo, H. A., Viveros-Bremauntz, F., Del-Val, E., Macías-Rodríguez, L., López-Carmona, D. A., Alarcón, A., et al. (2021). Alterations of foliar arthropod communities in a maize agroecosystem induced by the root-associated fungus Trichoderma harzianum. J. Pest. Sci. 94, 363–374. doi: 10.1007/s10340-020-01261-3
Das, P. P., Singh, K. R. B., Nagpure, G., Mansoori, A., Singh, R. P., Ghazi, I. A., et al. (2022). Plant-soil-microbes: a tripartite interaction for nutrient acquisition and better plant growth for sustainable agricultural practices. Environ. Res. 214:113821. doi: 10.1016/j.envres.2022.113821
de Chaves, M., Antunes, P. R., Souza, B., da Costa, R., de Paschoal, I., Teixeira, M. L., et al. (2022). Fungicide resistance in Fusarium graminearum species complex. Curr. Microbiol. 79, 1–9. doi: 10.1007/s00284-021-02759-4
Druzhinina, I. S., Seidl-Seiboth, V., Herrera-Estrella, A., Horwitz, B. A., Kenerley, C. M., Monte, E., et al. (2011). Trichoderma: the genomics of opportunistic success. Nat. Rev. Microbiol. 9, 749–759. doi: 10.1038/nrmicro2637
Elshafie, H. S., Sakr, S., Bufo, S. A., and Camele, I. (2017). An attempt of biocontrol the tomato-wilt disease caused by Verticillium dahliae using Burkholderia gladioli pv. agaricicola and its bioactive secondary metabolites. Int. J. Plant Biol. 8:7263. doi: 10.4081/pb.2017.7263
Elshahawy, I. E., and El-Mohamedy, R. S. (2019). Biological control of Pythium damping-off and root-rot diseases of tomato using Trichoderma isolates employed alone or in combination. J. Plant Pathol. 101, 597–608. doi: 10.1007/s42161-019-00248-z
Fishal, E. M., Mohd, I. B., Razak, A., Bohari, N. H., and Nasir, M. A. A. M. (2022). In vitro screening of endophytic Trichoderma sp. isolated from oil palm in FGV plantation against Ganoderma boninense. Adv. Microbiol. 12, 443–457. doi: 10.4236/aim.2022.127031
França, D. V., Cardozo, K. C., Kupper, M. M., Magri, R., Gomes, T. M., and Rossi, F. (2017). Trichoderma spp. isolates with potential of phosphate solubilization and growth promotion in cherry tomato. Pesqui. Agropec. Trop. 47, 360–368. doi: 10.1590/1983-40632017v4746447
Ghazala, I., Charfeddine, M., Charfeddine, S., Haddar, A., Ellouz-Chaabouni, S., and Gargouri-Bouzid, R. (2022). Lipopeptides from Bacillus mojavensis I4 confer induced tolerance toward Rhizoctonia solani in potato (Solanum tuberosum). Physiol. Mol. Plant Pathol. 121:101895. doi: 10.1016/j.pmpp.2022.101895
Glick, B. R. (2012). Plant growth-promoting bacteria: mechanisms and applications. Scientifica 2012:963401:15. doi: 10.6064/2012/963401
Haidukowski, M., Pascale, M., Perrone, G., Pancaldi, D., Campagna, C., and Visconti, A. (2005). Effect of fungicides on the development of Fusarium head blight, yield and deoxynivalenol accumulation in wheat inoculated under field conditions with Fusarium graminearum and Fusarium culmorum. J. Sci. Food Agric. 85, 191–198. doi: 10.1002/jsfa.1965
Harman, G. E. (2006). Overview of mechanisms and uses of Trichoderma spp. Phytopathology 96, 190–194. doi: 10.1094/PHYTO-96-0190
Harman, G. E., and Christian, P. K. (2002). Trichoderma and Gliocladium. Volume 1: Basic biology, taxonomy and genetics. CRC Press.
Harman, G. E., Howell, C. R., Viterbo, A., Chet, I., and Lorito, M. (2004). Trichoderma species–opportunistic, avirulent plant symbionts. Nat. Rev. Microbiol. 2, 43–56. doi: 10.1038/nrmicro797
Hermosa, R., Cardoza, R. E., Rubio, M. B., Gutiérrez, S., and Monte, E. (2014). “Secondary metabolism and antimicrobial metabolites of Trichoderma” in Biotechnology and Biology of Trichoderma. eds. I. Druzhinina and M. Tuohy (Amsterdam, Netherlands: Elsevier), 125–137.
Hermosa, R., Viterbo, A., Chet, I., and Monte, E. (2012). Plant-beneficial effects of Trichoderma and of its genes. Microbiology 158, 17–25. doi: 10.1099/mic.0.052274-0
Hernández-Aparicio, F., Lisón, P., Rodrigo, I., Bellés, J. M., and López-Gresa, M. P. (2021). Signaling in the tomato immunity against Fusarium oxysporum. Molecules 26:1818. doi: 10.3390/molecules26071818
Hewedy, O. A., Abdel, K. S., Lateif, M. F., Seleiman, A. S., Albarakaty, F. M., and El-Meihy, R. M. (2020b). Phylogenetic diversity of Trichoderma strains and their antagonistic potential against soil-borne pathogens under stress conditions. Biology 9:189. doi: 10.3390/biology9080189
Hewedy, O. A., Abdel-Lateif, K. S., and Bakr, R. A. (2020a). Genetic diversity and biocontrol efficacy of indigenous Trichoderma isolates against Fusarium wilt of pepper. J. Basic Microbiol. 60, 126–135. doi: 10.1002/jobm.201900493
Hewedy, O. A., El-Zanaty, A. M., and Fahmi, A. I. (2020c). “Screening and identification of novel cellulolytic Trichoderma species from Egyptian habitats.” BioTechnologia. Journal of Biotechnology Computational Biology and Bionanotechnology 101.
Irzykowska, L., Bocianowski, J., Waśkiewicz, A., Weber, Z., Karolewski, Z., Goliński, P., et al. (2012). Genetic variation of Fusarium oxysporum isolates forming fumonisin B1 and moniliformin. J. Appl. Genet. 53, 237–247. doi: 10.1007/s13353-012-0087-z
Jangir, M., Sharma, S., and Sharma, S. (2019). Target and non-target effects of dual inoculation of biocontrol agents against Fusarium wilt in Solanum lycopersicum. Biol. Control 138:104069. doi: 10.1016/j.biocontrol.2019.104069
Jones, J. B., Zitter, T. A., Momol, T. M., and Miller, S. A. (2014). Compendium of Tomato Diseases and Pests. United States: APS Press.
Kamaruzzaman, M., Islam, M. S., Mahmud, S., Polash, S. A., Razia Sultana, M., Hasan, A., et al. (2021). In vitro and in silico approach of fungal growth inhibition by Trichoderma asperellum HbGT6-07 derived volatile organic compounds. Arab. J. Chem. 14:103290. doi: 10.1016/j.arabjc.2021.103290
Karthika, K. S., Rashmi, I., and Parvathi, M. S. (2018). “Biological functions, uptake and transport of essential nutrients in relation to plant growth”, in Plant Nutrients and Abiotic Stress Tolerance. eds. M. Hasanuzzaman, M. Fujita, H. Oku, K. Nahar, and B. Hawrylak-Nowak (Singapore: Springer), 1–49.
Ketta, H. A., and Hewedy, O. A. E.-R. (2021). Biological control of Phaseolus vulgaris and Pisum sativum root rot disease using Trichoderma species. Egypt. J. Biol. Pest Control 31, 1–9. doi: 10.1186/s41938-021-00441-2
Khan, R. A. A., Najeeb, S., Mao, Z., Ling, J., Yang, Y., Li, Y., et al. (2020). Bioactive secondary metabolites from Trichoderma spp. against phytopathogenic bacteria and root-knot nematode. Microorganisms 8, 401–402. doi: 10.3390/microorganisms8030401
Kipngeno, P., Losenge, T., Maina, N., Kahangi, E., and Juma, P. (2015). Efficacy of Bacillus subtilis and Trichoderma asperellum against Pythium aphanidermatum in tomatoes. Biol. Control 90, 92–95. doi: 10.1016/j.biocontrol.2015.05.017
Lei, Z. H. A. O., and Zhang, Y.-q. (2015). Effects of phosphate solubilization and phytohormone production of Trichoderma asperellum Q1 on promoting cucumber growth under salt stress. J. Integr. Agric. 14, 1588–1597. doi: 10.1016/S2095-3119(14)60966-7
Li, Y.-T., Hwang, S.-G., Huang, Y.-M., and Huang, C.-H. (2018). Effects of Trichoderma asperellum on nutrient uptake and Fusarium wilt of tomato. Crop Prot. 110, 275–282. doi: 10.1016/j.cropro.2017.03.021
Liu, S., Ruan, W., Li, J., Xu, H., Wang, J., Gao, Y., et al. (2008). Biological control of phytopathogenic fungi by fatty acids. Mycopathologia 166, 93–102. doi: 10.1007/s11046-008-9124-1
López Errasquín, E., and Vázquez, C. (2003). Tolerance and uptake of heavy metals by Trichoderma atroviride isolated from sludge. Chemosphere 50, 137–143. doi: 10.1016/S0045-6535(02)00485-X
Lori, G., Sisterna, M., Haidukowski, M., and Rizzo, I. (2003). Fusarium graminearum and deoxynivalenol contamination in the durum wheat area of Argentina. Microbiol. Res. 158, 29–35. doi: 10.1078/0944-5013-00173
Maliehe, T. S., Mbambo, M., Nqotheni, M. I., Senzo, N. S., and Shandu, J. S. E. (2022). Antibacterial effect and mode of action of secondary metabolites from fungal endophyte associated with Aloe ferox Mill. Microbiol. Res. 13, 90–101. doi: 10.3390/microbiolres13010007
Manikandan, R., Harish, S., Karthikeyan, G., and Raguchander, T. (2018). Comparative proteomic analysis of different isolates of Fusarium oxysporum f. sp. lycopersici to exploit the differentially expressed proteins responsible for virulence on tomato plants. Front. Microbiol. 9:420. doi: 10.3389/fmicb.2018.00420
Marshall, H., Meneely, J. P., Quinn, B., Zhao, Y., Bourke, P., Gilmore, B. F., et al. (2020). Novel decontamination approaches and their potential application for post-harvest aflatoxin control. Trends Food Sci. Technol. 106, 489–496. doi: 10.1016/j.tifs.2020.11.001
Martínez-Medina, A., Appels, F. V. W., and van Wees, S. C. M. (2017). Impact of salicylic acid-and jasmonic acid-regulated defences on root colonization by Trichoderma harzianum T-78. Plant Signal. Behav. 12:e1345404. doi: 10.1080/15592324.2017.1345404
Marzano, M., Gallo, A., and Altomare, C. (2013). Improvement of biocontrol efficacy of Trichoderma harzianum vs. Fusarium oxysporum f. sp. lycopersici through UV-induced tolerance to fusaric acid. Biol. Control 67, 397–408. doi: 10.1016/j.biocontrol.2013.09.008
Meena, M., Swapnil, P., Zehra, A., Dubey, M. K., and Upadhyay, R. S. (2017). Antagonistic assessment of Trichoderma spp. by producing volatile and non-volatile compounds against different fungal pathogens. Arch. Phytopathol. Plant Protect. 50, 629–648. doi: 10.1080/03235408.2017.1357360
Michell, R. H. (2008). Inositol derivatives: evolution and functions. Nat. Rev. Mol. Cell Biol. 9, 151–161. doi: 10.1038/nrm2334
Modrzewska, M., Bryła, M., Kanabus, J., and Pierzgalski, A. (2022). Trichoderma as a biostimulator and biocontrol agent against Fusarium in the production of cereal crops: opportunities and possibilities. Plant Pathol. 71, 1471–1485. doi: 10.1111/ppa.13578
Mohiddin, F. A., Khan, M. R., Khan, S. M., and Bhat, B. H. (2010). Why Trichoderma is considered super hero (super fungus) against the evil parasites? Plant Pathol. J. 9, 92–102. doi: 10.3923/ppj.2010.92.102
Mukherjee, P. K., Mendoza-Mendoza, A., Zeilinger, S., and Horwitz, B. A. (2022). Mycoparasitism as a mechanism of Trichoderma-mediated suppression of plant diseases. Fungal Biol. Rev. 39, 15–33. doi: 10.1016/j.fbr.2021.11.004
Mulatu, A., Megersa, N., Tolcha, T., Alemu, T., and Vetukuri, R. R. (2022). Bioassay evaluation, GC-MS analysis and acute toxicity assessment of Methanolic extracts of Trichoderma species in an animal model. bioRxiv 2022:480498. doi: 10.1101/2022.02.15.480498
Munkvold, G. P. (2017). Fusarium species and their associated mycotoxins. Methods Mol. Biol. 154, 51–106. doi: 10.1007/978-1-4939-6707-0_4
Mwangi, M. W., Monda, E. O., Okoth, S. A., and Jefwa, J. M. (2011). Inoculation of tomato seedlings with Trichoderma harzianum and Arbuscular mycorrhizal fungi and their effect on growth and control of wilt in tomato seedlings. Braz. J. Microbiol. 42, 508–513. doi: 10.1590/S1517-83822011000200015
Nakai, J. (2018). Food and Agriculture Organization of the United Nations and the Sustainable Development Goals. Sustainable Development, No. 22.
Nandini, B., Puttaswamy, H., Saini, R. K., Prakash, H. S., and Geetha, N. (2021). Trichovariability in rhizosphere soil samples and their biocontrol potential against downy mildew pathogen in pearl millet. Sci. Rep. 11, 1–15. doi: 10.1038/s41598-021-89061-2
Natsiopoulos, D., Tziolias, A., Lagogiannis, I., Mantzoukas, S., and Eliopoulos, P. A. (2022). Growth-promoting and protective effect of Trichoderma atrobrunneum and T. simmonsii on tomato against soil-borne fungal pathogens. Crops 2, 202–217. doi: 10.3390/crops2030015
Nautiyal, C. S. (1999). An efficient microbiological growth medium for screening phosphate solubilizing microorganisms. FEMS Microbiol. Lett. 170, 265–270. doi: 10.1111/j.1574-6968.1999.tb13383.x
Olowe, O. M., Nicola, L., Asemoloye, M. D., Akanmu, A. O., and Babalola, O. O. (2022). Trichoderma: potential bio-resource for the management of tomato root rot diseases in Africa. Microbiol. Res. 257:126978. doi: 10.1016/j.micres.2022.126978
Pal, K. K., and Gardener, B. M. (2006). Biological Control of Plant Pathogens. St. Paul, MN: American Psychopathological Society.
Pande, A., Pandey, P., Mehra, S., Singh, M., and Kaushik, S. (2017). Phenotypic and genotypic characterization of phosphate solubilizing bacteria and their efficiency on the growth of maize. J. Genet. Eng. Biotechnol. 15, 379–391. doi: 10.1016/j.jgeb.2017.06.005
Pramanik, K., and Mohapatra, P. P. (2017). Role of auxin on growth, yield and quality of tomato-a review. Int. J. Curr. Microbiol. Appl. Sci 6, 1624–1636. doi: 10.20546/ijcmas.2017.611.195
Pritesh, P., and Subramanian, R. B. (2011). PCR based method for testing Fusarium wilt resistance of tomato. Afr. J. Basic Appl. Sci. 3:222.
Qualhato, T. F., Lopes, F. A. C., Steindorff, A. S., Brandão, R. S., Jesuino, R. S. A., and Ulhoa, C. J. (2013). Mycoparasitism studies of Trichoderma species against three phytopathogenic fungi: evaluation of antagonism and hydrolytic enzyme production. Biotechnol. Lett. 35, 1461–1468. doi: 10.1007/s10529-013-1225-3
Ribeiro, J. A., Albuquerque, A., Materatski, P., Patanita, M., Varanda, C. M. R., Félix, M. D. R., et al. (2022). Tomato response to Fusarium spp. infection under field conditions: study of potential genes involved. Horticulturae 8:433. doi: 10.3390/horticulturae8050433
Ristaino, J. B., Anderson, P. K., Bebber, D. P., Brauman, K. A., Cunniffe, N. J., Fedoroff, N. V., et al. (2021). The persistent threat of emerging plant disease pandemics to global food security. Proc. Natl. Acad. Sci. 118:e2022239118. doi: 10.1073/pnas.2022239118
Roitsch, T., Balibrea, M. E., Hofmann, M., Proels, R., and Sinha, A. K. (2003). Extracellular invertase: key metabolic enzyme and PR protein. J. Exp. Bot. 54, 513–524. doi: 10.1093/jxb/erg050
Ron, M., Dorrity, M. W., de Lucas, M., Ted Toal, R., Hernandez, I., Little, S. A., et al. (2013). Identification of novel loci regulating interspecific variation in root morphology and cellular development in tomato. Plant Physiol. 162, 755–768. doi: 10.1104/pp.113.217802
Ruano-Rosa, D., Prieto, P., Rincón, A. M., Gómez-Rodríguez, M. V., Valderrama, R., Barroso, J. B., et al. (2016). Fate of Trichoderma harzianum in the olive rhizosphere: time course of the root colonization process and interaction with the fungal pathogen Verticillium dahliae. BioControl 61, 269–282. doi: 10.1007/s10526-015-9706-z
Saengchan, C., Sangpueak, R., Le Thanh, T., Phansak, P., and Buensanteai, N. (2022). Induced resistance against Fusarium solani root rot disease in cassava plant (Manihot esculenta Crantz) promoted by salicylic acid and Bacillus subtilis. Acta Agric. Scand. B Soil Plant Sci. 72:526. doi: 10.1080/09064710.2021.2018033
Salas-Marina, M. A., Silva-Flores, M. A., Uresti-Rivera, E. E., Castro-Longoria, E., Herrera-Estrella, A., and Casas-Flores, S. (2011). Colonization of Arabidopsis roots by Trichoderma atroviride promotes growth and enhances systemic disease resistance through jasmonic acid/ethylene and salicylic acid pathways. Eur. J. Plant Pathol. 131, 15–26. doi: 10.1007/s10658-011-9782-6
Sallam, N., Eraky, A. M. I., and Sallam, A. (2019). Effect of Trichoderma spp. on Fusarium wilt disease of tomato. Mol. Biol. Rep. 46, 4463–4470. doi: 10.1007/s11033-019-04901-9
Scudeletti, D., Crusciol, C. A. C., Bossolani, J. W., Moretti, L. G., Momesso, L., Tubana, B. S., et al. (2021). Trichoderma asperellum inoculation as a tool for attenuating drought stress in sugarcane. Front. Plant Sci. 12:645542. doi: 10.3389/fpls.2021.645542
Selim, M. E., and El-Gammal, N. A. (2015). Role of fusaric acid mycotoxin in pathogensis process of tomato wilt disease caused by Fusarium oxysporum. J. Bioproc. Biotech. 5:1. doi: 10.4172/2155-9821.1000255
Sharma, A., Salwan, R., Kaur, R., Sharma, R., and Sharma, V. (2022). Characterization and evaluation of bioformulation from antagonistic and flower inducing Trichoderma asperellum isolate UCRD5. Biocatal. Agric. Biotechnol. 43:102437. doi: 10.1016/j.bcab.2022.102437
Sharon, E., Chet, I., Viterbo, A., Bar-Eyal, M., Nagan, H., Samuels, G. J., et al. (2007). Parasitism of Trichoderma on Meloidogyne javanica and role of the gelatinous matrix. Eur. J. Plant Pathol. 118, 247–258. doi: 10.1007/s10658-007-9140-x
Siddiquee, S., Cheong, B. E., Taslima, K., Kausar, H., and Hasan, M. M. (2012). Separation and identification of volatile compounds from liquid cultures of Trichoderma harzianum by GC-MS using three different capillary columns. J. Chromatogr. Sci. 50, 358–367. doi: 10.1093/chromsci/bms012
Simons, M., Van Der Bij, A. J., Brand, I., De Weger, L. A., Wijffelman, C. A., and Lugtenberg, B. J. (1996). Gnotobiotic system for studying rhizosphere colonization by plant growth-promoting pseudomonas bacteria. Mol. Plant Microbe Interact. 9, 600–607. doi: 10.1094/MPMI-9-0600
Srivastava, R., Khalid, A., Singh, U. S., and Sharma, A. K. (2010). Evaluation of arbuscular mycorrhizal fungus, fluorescent pseudomonas and Trichoderma harzianum formulation against Fusarium oxysporum f. sp. lycopersici for the management of tomato wilt. Biol. Control 53, 24–31. doi: 10.1016/j.biocontrol.2009.11.012
Stracquadanio, C., Luz, C., La Spada, F., Meca, G., and Cacciola, S. O. (2021). Inhibition of Mycotoxigenic fungi in different vegetable matrices by extracts of Trichoderma species. J. Fungi (Basel) 7:445. doi: 10.3390/jof7060445
Su, S., Zeng, X., Bai, L., Jiang, X., and Li, L. (2010). Bioaccumulation and biovolatilisation of pentavalent arsenic by Penicillin janthinellum, Fusarium oxysporum and Trichoderma asperellum under laboratory conditions. Curr. Microbiol. 61, 261–266. doi: 10.1007/s00284-010-9605-6
Taylor, J. T., Krieger, I., Crutcher, F. K., Jamieson, P., Horwitz, B. A., Kolomiets, M. V., et al. (2021). A class I hydrophobin in Trichoderma virens influences plant-microbe interactions through enhancement of enzyme activity and MAMP recognition. bioRxiv 2021:425738. doi: 10.1101/2021.01.07.425738.
Valluru, R., and Van den Ende, W. (2011). Myo-inositol and beyond–emerging networks under stress. Plant Sci. 181, 387–400. doi: 10.1016/j.plantsci.2011.07.009
Walters, D., Raynor, L., Mitchell, A., Walker, R., and Walker, K. (2004). Antifungal activities of four fatty acids against plant pathogenic fungi. Mycopathologia 157, 87–90. doi: 10.1023/B:MYCO.0000012222.68156.2c
Waskiewicz, A., Golinski, P., Karolewski, Z., Irzykowska, L., Bocianowski, J., Kostecki, M., et al. (2010). Formation of fumonisins and other secondary metabolites by Fusarium oxysporum and F. proliferatum: a comparative study. Food Addit. Contam. 27, 608–615. doi: 10.1080/19440040903551947
White, T. J., Bruns, T. D., Lee, S. B., and Taylor, J. W. (1990). Amplification and direct sequencing of fungal ribosomal RNA genes for phylogenetics. PCR Protoc. 18, 315–322. doi: 10.1016/B978-0-12-372180-8.50042-1
Wiedemeier, A. M. D., Judy-March, J. E., Hocart, C. H., Wasteneys, G. O., Williamson, R. E., and Baskin, T. I. (2002). Mutant alleles of Arabidopsis RADIALLY SWOLLEN 4 and 7 reduce growth anisotropy without altering the transverse orientation of cortical microtubules or cellulose microfibrils. Development 129, 4821–4830. doi: 10.1242/dev.129.20.4821
Woldeamanuale, T. (2017). Isolation, screening and identification of cadmium tolerant fungi and their removal potential. J. Forensic Sci. Crim. Investig. 5:555656. doi: 10.19080/JFSCI.2017.05.555656
Wu, Q., Sun, R., Ni, M., Jia, Y., Li, Y., Chuanjin, Y., et al. (2017). Identification of a novel fungus, Trichoderma asperellum GDFS1009, and comprehensive evaluation of its biocontrol efficacy. PLoS One 12:e0179957. doi: 10.1371/journal.pone.0179957
Yoo, S.-J., Shin, D. J., Won, H. Y., Song, J., and Sang, M. K. (2018). Aspergillus terreus JF27 promotes the growth of tomato plants and induces resistance against Pseudomonas syringae pv. Tomato. Mycobiology 46, 147–153. doi: 10.1080/12298093.2018.1475370
Zhalnina, K., Louie, K. B., Hao, Z., Mansoori, N., Nunes, U., da Rocha, S., et al. (2018). Dynamic root exudate chemistry and microbial substrate preferences drive patterns in rhizosphere microbial community assembly. Nat. Microbiol. 3, 470–480. doi: 10.1038/s41564-018-0129-3
Zhang, Y., and Ma, L.-J. (2017). Deciphering pathogenicity of Fusarium oxysporum from a phylogenomics perspective. Adv. Genet. 100, 179–209. doi: 10.1016/bs.adgen.2017.09.010
Keywords: Trichoderma asperellum, tomato, Fusarium oxysporum, IAA, biological control, root anatomy, confocal microscopy
Citation: Sehim AE, Hewedy OA, Altammar KA, Alhumaidi MS and Abd Elghaffar RY (2023) Trichoderma asperellum empowers tomato plants and suppresses Fusarium oxysporum through priming responses. Front. Microbiol. 14:1140378. doi: 10.3389/fmicb.2023.1140378
Edited by:
George Newcombe, University of Idaho, United StatesReviewed by:
Debdulal Banerjee, Vidyasagar University, IndiaShaikhul Islam, Bangladesh Agricultural Research Council, Bangladesh
Copyright © 2023 Sehim, Hewedy, Altammar, Alhumaidi and Abd Elghaffar. This is an open-access article distributed under the terms of the Creative Commons Attribution License (CC BY). The use, distribution or reproduction in other forums is permitted, provided the original author(s) and the copyright owner(s) are credited and that the original publication in this journal is cited, in accordance with accepted academic practice. No use, distribution or reproduction is permitted which does not comply with these terms.
*Correspondence: Omar A. Hewedy, hewedy.omar@gmail.com
†ORCID: Amira E. Sehim, https://orcid.org/0000-0003-4001-2009
Omar A. Hewedy, https://orcid.org/0000-0001-6764-9566
Khadijah A. Altammar, https://orcid.org/0000-0002-8691-086X
Rasha Y. Abd Elghaffar, https://orcid.org/0000-0003-4829-1751